Pushing the limits of protein expression with miRNAs
Posted: 17 March 2016 | Kerstin Otte Biberach (University of Applied Sciences), René Handrick (University of Applied Sciences), Simon Fischer (Boehringer Ingelheim) | No comments yet
Biopharmaceuticals are highly complex protein drugs such as antibodies, cytokines and peptide hormones, which are used for a variety of indication areas, including immunology, neurology, metabolic diseases and cancer. These modern protein drugs may hinder progression of a disease, ease symptoms or assure – in the case of chronic diseases – the long-term quality of patients’ lives.
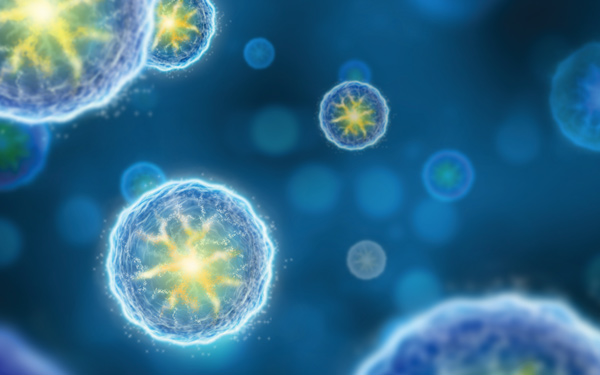
The percentage of the overall pharmaceutical drug pipeline that comprises biopharmaceuticals is significantly expanding and the demand for biologics is expected to further increase within the coming decades1 . In addition, as patents are nearing expiry, the production of biosimilars, also known as follow-on biologics or subsequent entry biologics, which are almost identical copies of an original product, is gaining increasing importance2 . This growth in the biopharmaceutical space makes it important to steadily improve manufacturing capacities to ensure market supply.
Protein production using mammalian cell systems For the production of biopharmaceuticals, mammalian cell-based production platforms constitute the most widespread expression systems. Production cells are genetically modified to produce the desired therapeutic protein and are subsequently cultured in large bioreactors where the cells secrete the translated proteins into the production medium. However, mammalian expression systems still exhibit bottlenecks compared with bacterial or yeast-based production hosts regarding growth capacity, cultivation time and product yield.
Chinese hamster ovary (CHO) cells represent the most frequently used mammalian production system for therapeutic proteins due to several key advantages over other cell types3 . Their robust growth in chemically-defined and serum-free suspension culture, a reasonable safety profile regarding human pathogenic virus replication, as well as their ability to express recombinant proteins with human-like posttranslational modifications, make them superior production hosts. In addition, a variety of CHO cell lines are available that are easy to genetically modify and allow for straightforward strategies to integrate the gene of interest into the host cell genome via site-specific or random integration which is often followed by gene amplification strategies4 . However, although bioprocess and culture media optimisations have led to great achievements in terms of cell growth and production yields over the past two decades, there are still drawbacks such as expensive media formulations, moderate growth capacities and comparably low volumetric productivities. Furthermore, recombinant proteins derived from CHO cells were shown to sometimes be immunogenic since glycosylation patterns are not a hundred percent identical to that of humans.
For decades, these disadvantages have encouraged researchers to steadily improve manufacturing cells by host cell engineering5. These efforts comprised either the stable introduction of genes or knockout/knock-down of protein coding genes to increase cellular performance and yielded CHO cell derivatives that outperformed their parental cell lines regarding cell growth, apoptosis resistance, metabolism, productivity or the ability to express therapeutic proteins with tailored glycosylation profiles5. However, all these approaches aim at modifying single genome loci and may therefore induce limited phenotypic effects on the host cell line. In addition, overexpression of single genes may overcharge the translational machinery of the production cells which should predominantly serve to produce the desired therapeutic proteins in large quantities. Hence, genetic engineering represents a powerful tool to significantly increase efficiency of CHO cell-based production processes but current technologies may be limited in pushing the cells to maximal cellular production capacities.
MicroRNAs as engineering tools to improve protein production
Recently, overexpression of specific microRNAs (miRNAs) has been suggested to be highly useful for metabolic engineering of CHO production cells since miRNAs do not add translational burden to the cells compared with overexpression of advantageous engineering proteins6-8. MiRNAs are evolutionarily conserved small non-coding RNAs (ncRNAs) that post-transcriptionally modulate gene expression in eukaryotic cells9. They function as immediate response molecules to external stimuli and individual miRNAs can regulate multitudes of different genes concomitantly by imperfectly binding to specific target sites on the messenger RNA (mRNA), predominantly in the 3’-untranslated region (3’UTR).
First identified in 1993 as critical regulators of development in the nematode C.elegans10,11, miRNAs have been demonstrated to play essential roles in the regulation of virtually all cellular process in metazoans. Strikingly, one of the most interesting features of miRNAs is their extraordinarily high evolutionary conservation, which highlights their fundamental role in the regulation of cellular processes12.
We therefore set out to examine the potential of miRNAs as novel tools for next-generation cell engineering of biopharmaceutical production. However, the notion that there are currently 2,000 miRNAs known in the rodent genome makes the identification of effective miRNAs for cell line optimisation highly laborious. In order to identify miRNAs influencing cellular parameters which are critical for bioprocessing, we developed a comprehensive functional high-content miRNA screen in a recombinant CHO-SEAP cell line employing an entire murine miRNA mimics library comprising 1,139 different miRNAs13,14. The screening procedure comprised transient transfection of the entire miRNA library connected to a multi-parametric cellular readout based on flow cytometry. We were thereby able to unravel miRNA-mediated influences on a variety of bioprocess-relevant cellular parameters such as cell proliferation, recombinant protein expression, apoptosis and necrosis. Changes in SEAP protein levels were quantified by a chemiluminescent SEAP reporter gene assay.
Taking advantage of this high-throughput and high-content screening procedure, we were able to identify a huge number of miRNAs (in total 754 miRNAs or 66% of the miRNA library) to significantly affect the above-mentioned CHO cell phenotypes15. Intriguingly, we found that for each readout parameter, approximately 25-30% of the miRNA library accounted for statistically significant changes in the respective phenotype (Table 1; page 00). Regarding SEAP productivity, 185 transfected miRNAs significantly increased SEAP yields in the supernatant after three days with the best candidates showing an improvement of up to twofold. Significantly elevated cell-specific productivity was even detected for 238 miRNAs and significantly higher VCD was determined for 53 miRNAs, whereas 146 miRNAs decreased the apoptosis rate. The percentage of miRNAs boosting cell proliferation was in line with the fact that with the fact that 42 miRNAs decreased the number of necrotic cells indicating higher viabilities following miRNA introduction.
Functionality of these impactful miRNAs was confirmed in validation experiments by re-transfecting miRNAs in different CHO cell lines expressing various recombinant proteins. For instance, 92% of the pro-productive miRNAs could be successfully validated to enhance cellular productivity after transient miRNA mimics transfection CHO cells. This already indicated that the majority of our identified effective miRNAs may function independently of the cellular background as well as the product which is produced by the cell factories.
MiR-30 overexpression increases protein production
Besides the large number of identified target miRNAs in transient transfection experiments, we discovered that the entire miR-30 gene family substantially improves bioprocess performance of CHO cells14. A characteristic feature of an miRNA family is that the mature miRNA strands share a common ‘seed’ sequence which perfectly base pairs with the 3’UTR on its target mRNA. Out of the miR-30 family, we selected miR-30a, miR-30c and miR-30e to confirm that results of transient introduction of miRNA mimics can be extrapolated to stable miRNA overexpression.
For stable long-term expression of target miRNAs the respective precursor sequence has to be integrated into the host cell genome. Thus miRNA-precursor sequences were cloned into expression vectors and stable overexpressing cell pools based on the CHO-SEAP and also CHO-Antibody expressing parental cell lines were established. After batch cultivation of all three stable miRNA cell lines, analysis of SEAP protein concentration in the supernatant confirmed that all cell pools produced significantly more SEAP or antibody as compared with control cells. In subsequent experiments we could show that miR-30a overexpressing cells reached far higher cell density and viability and thereby increased volumetric productivity. In contrast, miR-30c overexpressing pools showed slightly decreased cell concentrations, however, cell-specific SEAP or antibody productivity was substantially increased.
In summary, stable miR-30 overexpressing cells clearly outperformed the parental cell line and revealed that elevated protein titers were achieved either by enhanced specific productivity or by increased viable cell density14. Strikingly, we demonstrate that members of the same miRNA family can have a remarkably diverse impact on cell function in CHO cells by targeting various cellular pathways. These results highlight again that miRNAs are attractive tools for improving culture performance of CHO cells.
Identification of underlying mechanisms of action
Finally, finding and validating potential target genes for selected impactful miRNAs might enable the identification of biological pathways that are crucial for enhanced cellular protein production induced by the overexpression of miRNAs.
In order to better understand the biological activity of miR-30 in CHO cells leading to increased productivity, we aimed to unravel potential target genes which are post-transcriptionally regulated by the miR-30 family in CHO cells. Therefore, we set out to identify putative target genes of the entire miR-30 family using bioinformatics tools. Determination of overlapping target genes and subsequent functional clustering revealed that 11 target genes could be clustered into three distinct subgroups, among them the ubiquitin pathway16.
The ubiquitin proteasome pathway (UPP) is the principal mechanism for protein catabolism in the eukaryotic cell. Degradation of a protein via the UPP involves two successive steps of tagging the substrate protein by the covalent attachment of multiple ubiquitin molecules and subsequent degradation of the tagged protein by the proteasome. This classical function of ubiquitin is associated with, for example, housekeeping functions or regulation of protein turnover. The attachment of ubiquitin to the target proteins requires a series of ATP-dependent enzymatic steps by E1 ubiquitin-activating enzymes, E2 ubiquitin-conjugating enzymes and E3 ubiquitin-ligating enzymes. Our in silico analyses revealed that the miR-30 family may regulate the ubiquitin pathway in CHO cells by targeting the ubiquitin-conjugating enzyme (Ube2j1) and the F-box protein termed S-phase kinase-associated protein 2 (Skp2)16.
Comprehensive functional validations showed that miR-30 overexpression downregulates both Ube2j1 and Skp2 mRNA expression. To elucidate whether miR-30 directly regulates any of these ubiquitin pathway genes, we performed luciferase reporter assays and were able to show that the miR-30 family does indeed directly bind to Skp2 mRNA and thereby downregulates its expression16. In addition, siRNA-mediated knock-down of Skp2 in CHO-SEQP cells actually led to an increase in cell-specific SEAP productivity and could successfully phenocopy pro-productive effects of miR-30 family members in CHO cells.
Skp2 is the substrate recognition component of the SCF (SKP1-CUL1-F-box protein) E3 ubiquitin-protein ligase complex which mediates the ubiquitination of target proteins, whereas Ube2j1 catalyses the covalent attachment of ubiquitin to other proteins (Figure 1; page 00). Inhibition of Skp2 and UBE2J1 by miR-30 and thereby polyubiquitinylation of target proteins may influence cellular processes such as DNA repair, apoptosis or endocytosis of cell surface receptors and finally lead to enhanced protein expression.
In summary, these results suggest that the miR-30 family regulates the ubiquitin pathway in CHO cells by targeting the ubiquitin E3 ligase Skp2 and likely also the ubiquitin-conjugating enzyme Ube2j1, exerting E2 ligase activity. The ubiquitin pathway represents an extremely interesting pathway in the context of protein production, as in yeast it is already known to increase productivity. Ubiquitination represents a tightly organised post-translational modification step to tag endogenous proteins for degradation or activation, depending on the type of ubiquitination. This allows a cell to manage the proteomic balance and to react to internal or external stimuli. The regulation of the ubiquitin pathway by overexpression of the miR-30 family may therefore shift the balance towards a high-producing phenotype while compromising biomass production in the fermentation process. Our results highlight the application of miRNAs as powerful tools for cell engineering and will shed additional light on these tiny but mighty regulators of gene expression.
References
- Zhu J. Mammalian cell protein expression for biopharmaceutical production. Biotechnology advances. 2012;30(5):1158-70
- Tsuruta LR, Lopes dos Santos M, Moro AM. Biosimilars advancements: Moving on to the future. Biotechnology progress. 2015;31(5):1139-49
- Kim JY, Kim YG, Lee GM. CHO cells in biotechnology for production of recombinant proteins: current state and further potential. Applied microbiology and biotechnology. 2012;93(3):917-30
- Wurm FM. Production of recombinant protein therapeutics in cultivated mammalian cells. Nature biotechnology. 2004;22(11):1393-8
- Fischer S, Handrick R, Otte K. The art of CHO cell engineering: A comprehensive retrospect and future perspectives. Biotechnology advances. 2015;33(8):1878-96
- Hackl M, Borth N, Grillari J. miRNAs–pathway engineering of CHO cell factories that avoids translational burdening. Trends in biotechnology. 2012;30(8):405-6
- Stiefel F, Fischer S, Hackl M, Handrick R, Hesse F, Borth N, et al. Noncoding RNAs, post-transcriptional RNA operons and Chinese hamster ovary cells. Pharmaceutical Bioprocessing. 2015;3(3):227-47
- Jadhav V, Hackl M, Druz A, Shridhar S, Chung CY, Heffner KM, et al. CHO microRNA engineering is growing up: Recent successes and future challenges. Biotechnology advances. 2013
- Bartel DP. MicroRNAs: target recognition and regulatory functions. Cell. 2009;136(2):215-33
- Lee RC, Feinbaum RL, Ambros V. The C. elegans heterochronic gene lin-4 encodes small RNAs with antisense complementarity to lin-14. Cell. 1993;75(5):843-54
- Wightman B, Ha I, Ruvkun G. Posttranscriptional regulation of the heterochronic gene lin-14 by lin-4 mediates temporal pattern formation in C. elegans. Cell. 1993;75(5):855-62
- Gurtan AM, Sharp PA. The Role of miRNAs in Regulating Gene Expression Networks. Journal of molecular biology. 2013
- Fischer S, Wagner A, Kos A, Aschrafi A, Handrick R, Hannemann J, et al. Breaking limitations of complex culture media: functional non-viral miRNA delivery into pharmaceutical production cell lines. Journal of biotechnology. 2013;168(4):589-600
- Fischer S, Buck T, Wagner A, Ehrhart C, Giancaterino J, Mang S, et al. A functional high-content miRNA screen identifies miR-30 family to boost recombinant protein production in CHO cells. Biotechnology journal. 2014;9(10):1279-92
- Fischer S, Handrick R, Aschrafi A, Otte K. Unveiling the principle of microRNA-mediated redundancy in cellular pathway regulation. RNA biology. 2015;12(3):238-47
- Fischer S, Mathias S, Schaz S, Emmerling VV, Buck T, Kleemann M, et al. Enhanced protein production by microRNA-30 family in CHO cells is mediated by the modulation of the ubiquitin pathway. Journal of biotechnology. 2015;212:32-43
Biographies
Simon Fischer studied Pharmaceutical Biotechnology (BSc) at the University of Applied Sciences Biberach, Germany, and Biomedical Engineering (MSc) at the University of Applied Sciences Albstadt-Sigmaringen, Germany. After industrial engagements at the Department of CNS Research at Boehringer Ingelheim Pharma, Biberach, Germany, and the Novartis Institutes for BioMedical Research, Basel, Switzerland, he obtained his PhD in Pharmaceutical Biotechnology from Ulm University, Germany. Since 2015, Simon has worked as a Scientist at the Department of Biopharmaceutical Process Development at Boehringer Ingelheim Pharma, Biberach, Germany, where he is responsible for the development and implementation of novel technologies in cell line development.
René Handrick studied Biology at the University of Göttingen, Germany, and obtained his PhD at the Center of Biochemical Engineering, University of Stuttgart. After postdoctoral fellowship at the Department of Experimental Radiation Oncology, University of Tübingen, and at the Institute for Cell Biology, University of Essen, working on preclinical research projects, he went to the University of Applied Sciences Biberach, Germany, Faculty of Pharmaceutical Biotechnology. In his function as Scientist and Lab Head of the Institute of Applied Biotechnology he is involved in several applied as well as basic research projects focused on bioprocess and cell line development for the production of biopharmaceuticals.
Kerstin Otte is a Biologist trained from the Phillipps-University of Marburg, Germany, and she obtained her PhD at the SL-University in Uppsala, Sweden. After a postdoctoral fellowship at the Gurdon Institute at Cambridge, UK, she gained industry experience as Senior Scientist at Graffinity Bioscience and LION Bioscience, both Heidelberg, Germany, in preclinical drug development. Since 2006, Kerstin Otte has held a Professorship for Molecular and Cell Biology at the University of Applied Sciences Biberach, Germany, at the Faculty of Pharmaceutical Biotechnology. Her research focusses on cell line development for the production of biopharmaceuticals.
Related topics
miRNAs, Protein Expression
Related organisations
Boehringer Ingelheim, University of Applied Sciences
Related people
Kerstin Otte Biberach, René Handrick, Simon Fischer