Tackling the spread of pathogens in transport hubs
Posted: 13 December 2016 | Ilpo Kulmala (VTT Ltd) | No comments yet
Ilpo Kulmala, Principal Scientist at VTT Ltd, explains the role that transportation plays in the spread of infectious diseases…
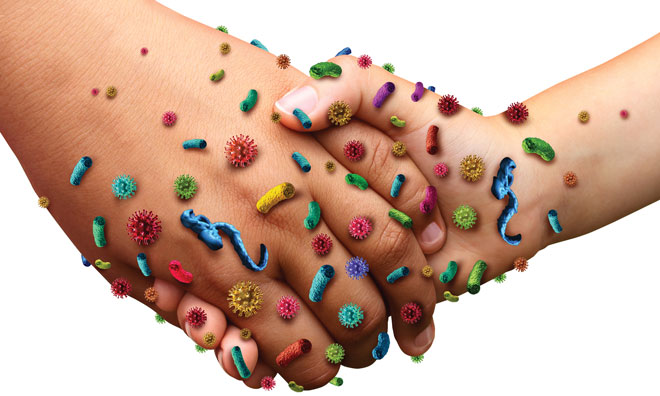
Whether naturally or intentionally released person-to-person transmissible, pathogens are spread. As was the case for severe acute respiratory syndrome (SARS) in 2002/03 and the influenza A(H1N1)pdm09 virus in early 2009, air travel is key to the spreading of such pathogens internationally. In addition, mass transportation systems offer an effective way of accelerating the spread of infectious diseases within communities, which is especially a problem during the rush hour period, when there is a high crowd density and thus contact rate. With transportation stops frequent and crowd turnover higher than normal at each stop, the pool of potential new susceptible subjects is frequently refreshed.
Sneezing, coughing, talking or even tidal breathing produces large numbers of pathogenic respiratory viruses that expose nearby passengers. In addition, commonly touched surfaces may be contaminated with pathogens, and microbes from these surfaces can be transferred to other persons’ hands and thereafter to portals of entry into the body (mucosal surfaces of the eyes, nose and mouth) when touching the face.
In the course of a European Union-funded project, Prevention and Management of High Threat Pathogen Incidents in Transport Hubs (PANDHUB), which was launched in 2014, a scoping review has been undertaken to identify surveillance systems and data sources that may be useful in detecting communicable disease public health events (including new or emerging infections and other high-threat pathogen incidents) in transport hubs of air, sea, rail and ground transport. Travel or transport feature in reports of outbreaks of new and emerging or re-emerging infections with epidemic or pandemic potential.
However, the evidence of transmission of infection at hubs (or during on-board transport) is sparse, partly owing to the difficulty of attributing exposure and transmission to a particular environment and the absence of experimental studies. That said, as travel contributed to the global spread of SARS and pandemic influenza and, with respect to MERS-CoV, primary cases have appeared in those travellers returning from the Middle East. Likewise, sporadic cases of Ebola virus disease (EVD) have been introduced in the European Union via returning healthcare workers and wider importation through travel has been an important concern throughout the recent crisis.
Hot spots
Although the risk of infection in transport hubs is low under normal conditions, the situation may change during pandemics or high-threat pathogenic incidents. In order to identify the areas of elevated risk for infection, hot spots in transport hubs have been identified. The hot spots are defined as points or sites within a traffic hub environment where the risk of microbial transmission is at least periodically increased due to favourable conditions or human behaviour. The transmission mechanisms considered in this context are direct and indirect contact, and airborne or droplet transmission, which are the main routes of infection of the diseases discussed, having differing potential challenges to health authorities.
Depending on the specific properties of a microbe, the potential sites for hot spots within a hub will vary in accordance with the microbe. For example, influenza’s potential hot spots, at least periodically, are formed by a high density of passengers, as well as frequently touched surfaces.
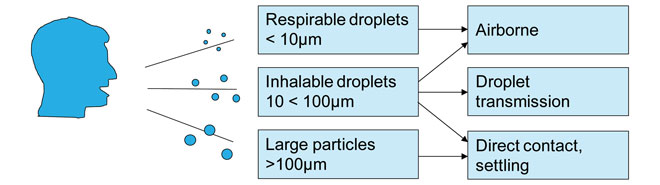
Figure 1: Schematic of respiratory disease transmission modes
Contact versus droplet transmission
Direct contact transmission occurs when microorganisms are transferred from one infected person to another without a contaminated intermediate object or person, while indirect contact transmission involves the transfer of an infectious agent through a contaminated intermediate object or person1. An example of direct contact is contact with blood or other body fluids through breaks in skin, or contact with a mucous membrane. Examples of indirect contact are the transfer of infection through touching a contaminated object and then self-inoculating a mucosal surface such as the nose, or use of contaminated equipment. In general, for microbes that transmit via direct contact to body fluids, direct or indirect contact with a surface is an important transmission route. Contaminated surfaces are present in, for example, lavatories and the touch screens of ticket or self-check-in machines, and certain other frequently touched surfaces like handrails.
Droplet transmission occurs when pathogen-laden particles are emitted from an infected host by sneezing, coughing or exhaling. These respiratory droplets transmit infections when they travel directly from the respiratory tract of an infected individual to a susceptible mucosal surface (in the eyes, nose, mouth and respiratory tract). Many infectious diseases are believed to be transmitted between individuals by airborne routes and via the large droplets produced by sneezing and coughing.
The behaviour of these aerosols depends on their size; coughs contain particles ranging from <1 to 2000μm in size, however, 99.9% are >10μm in diameter2 (Figure 1). Whilst large droplets will be heavily laden with virus, most of them will not be inhalable due to their size.
The infectious agents within the droplets remain infective over time and can be suspended in the air until removed by ventilation, however, because droplet nuclei are more concentrated nearer their source, they are more likely to transmit over short distances than long3. Indeed, historically, HICPAC suggested droplets were only transmitted over distances of less than three feet. However, in the light of evidence from SARS, this was reconsidered and the guideline was amended to state that pathogens transmitting via the droplet route do not remain infective over ‘long distances’, recommending face masks when within 6-10 feet for protection against infections transmitted via this route.
When they reach the body, droplet nuclei 5μm or less in diameter can penetrate the lungs as far as the alveolar region. Particles that can reach this point are termed ‘respirable’; those breathed in through the nose and mouth are termed ‘inhalable’. The respirable particles reaching deeper places in the lungs may be more potent in initiating infection, and a lower inoculum may be needed than with particles depositing in the upper airways.
Location of hot spots
The modes and therefore sources of transmission vary by the microbes causing infections. As well as the infection scenarios selected for further development in the PANDHUB project, the hot spots are relevant for other microbes and infections, which share their transmission modes and survival on surfaces with the model scenario infections.
The most important hot spots in transport hubs for the microbes transmitted by indirect contact are surfaces frequently touched by passengers, such as touch screens of self-service automats; security control boxes for carry-on luggage transfer; waiting halls and lavatories and the passport control self-service automats. In general, viruses and bacteria survive for longer on non-porous, water resistant surfaces, such as stainless steel and plastics, than on porous surfaces, such as fabrics and tissues (Table 1). In a study by Rusin et al. (2002) the transfer efficiencies of microbes from fomites to hands were found to be 26-65% for hard surfaces, compared with <0.01% from porous surfaces. In addition to the surface type, temperature and relative humidity have the greatest effects regarding survival on surfaces4.
In order for infection to be transmitted through indirect contact transmission, high titres of pathogen in excess of the infectious dose must be shed; the deposited pathogen must survive on surfaces; high titres must be collected via the hands; the pathogen must survive on hands and the hands must deposit an infectious dose of pathogen on target cells (usually mucous surfaces in the head).
Depending on the virus or bacterium, survival time may vary on the skin of hands: for example, up to 15 minutes with influenza A5 and for norovirus as much as several hours4. Meanwhile, it has been observed that people on the metro system touched their faces an average of 3.6 times per hour and common objects an average of 3.3 times per hour6. This rate of self-touching means that people are likely to get germs on their hands much more frequently than they wash germs off their hands, and many of the microbes survive long enough to allow transmission. Transfer efficiency of bacteria from the fingertip to the lip is similar to that observed from hard surfaces to hands8.
For the microbes transmitted by airborne or droplet routes, traffic hub areas with a high volume and density of passengers are the hot spots for potential contamination, such as station platforms or gates and arrival halls; baggage claim halls; border control; security control and custom inspection points. Figure 2 illustrates the identified hot spots for droplet and indirect contact transmission in a metro system. Many characteristics of the hot spots are identical or similar to the hubs in different traffic modes. However, the differences in passenger density and the time or speed of passenger flow between the different hub types will affect both the intensity and the duration of exposure, particularly for droplet transmission.
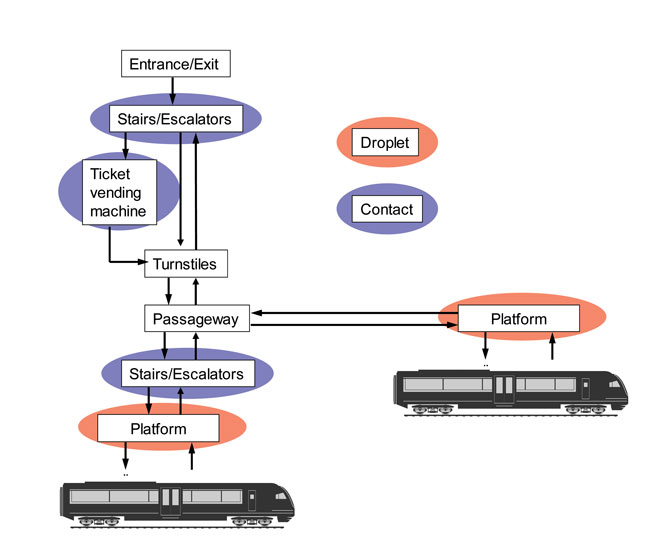
Figure 2: Passenger processes in metro system and potential hot spots for disease transmission
The ventilation system of transport hubs may contribute to the spread of naturally occurring or intentionally spread pathogens. How effectively this occurs depends on the characteristics of the HVAC system, for example, air distribution, flow rates and filtration efficiency, as well as the ventilated spaces. In general, confined and crowded spaces with inadequate ventilation increases the risk for airborne infection regardless of the mode of transport.
Conclusions
In summary, it can be concluded that a major factor in the transmission of infection is the mixing of infectious and susceptible individuals. A recent review study by Public Health England7 on the impact of mass gatherings on influenza pandemics concluded that the crowd density and event duration may be the key characteristics determining the risk of influenza transmission. Although the duration of mass gathering events is typically longer than residence times in transport hubs, the strong mixing and close proximity of passengers to each other, especially during peak hours, may increase disease transmission in confined spaces.
It is important to understand the basic mechanisms through which diseases are transmitted, in order to focus protective and preventive actions effectively. Recommendations issued to the public typically emphasise hand-washing, but during potentially severe disease outbreaks, the messages should be shifted to ensure that people understand how self-inoculation occurs and avoid touching their faces.
Many respiratory diseases have been found to have higher transmission rates when susceptible individuals are in close proximity to the infectious person: up to about 1-2m. Within this short range, the susceptible person has a substantially greater exposure from the directed respiratory spray consisting of both inspirable large droplets and respirable aerosols, thus maximising inhalation exposure to small particles and inspirable droplets.
The PANDHUB project will produce guidance for preparedness against pandemics and other high-threat pathogen incidents, taking into consideration the specific features of transport hubs.
Acknowledgements
This project has received funding from the European Union’s Seventh Framework Programme for research, technological development and demonstration under grant agreement no 607433.
ILPO KULMALA is a Principal Scientist at VTT Ltd. He has over 30 years’ experience in the field of ventilation, airborne contaminant transport modelling and exposure control as well as experimental validation of the simulations. His recent activities include research and development of chemical, biological and radiological (CBR) threat mitigation systems and protection of people and critical infrastructures against CBR threats. He has hands-on experience in research and development, inventing, developing and implementing new products related to ventilation, air cleaning and decontamination. He is currently the coordinator of the EU funded PANDHUB project aiming at prevention and management of pandemics and high threat pathogen incidents in transport hubs.
References
- Siegel JD, Rhinehart E, Jackson M, Chiarello L and the Healthcare Infection Control Practices Advisory Committee, 2007. Guideline for Isolation Precautions: Preventing Transmission of Infectious Agents in Healthcare Settings http://www.cdc.gov/ncidod/dhqp/pdf/isolation2007.pdf
- Papineni R, Rosenthal F. The size distribution of droplets in the exhaled breath of healthy human subjects. J Aerosol Med. 1997; 10:105-116
- Killingley B, Enstone J, Booy R, Hayward A, Oxford J, Ferguson N, et al. Potential role of human challenge studies for investigation of influenza transmission. Lancet Infect Dis. 2011; 11(11):879-886
- Rönnqvist M. 2014. Noroviruses on surfaces: Detection, transfer and inactivation. Academic dissertation. University of Helsinki
- Greatorex JS, Digard P, Curran MD, Moynihan R, Wensley H, Wreghitt T, Varsani H, Garcia F, Enstone J, Nguyen-Van-Tam JS. 2011. Survival of Influenza A(H1N1) on Materials Found in Households: Implications for Infection Control
- Alonso W, Nascimento FC, Shapiro J, Schuck-Paim C. Facing Ubiquitous Viruses: When Hand Washing Is Not Enough. Correspondence, Clinical Infectious Diseases. 2013; 56:617
- Department of Health DH 2014. Impact of Mass Gatherings on an Influenza Pandemic. Public Health England
- Rusin P, Maxwell S, Gerba C. Comparative surface-to-hand and fingertip-to-mouth transfer efficiency of gram-positive bacteria, gram-negative bacteria, and phage. Journal of Applied Microbiology. 2002; 93, 585–592.
Related topics
Pathology & Molecular Medicine