Marine Drug Discovery: Realising the potential of therapeutics from marine sources – lessons from Big Pharma
Posted: 3 May 2014 | Marcel Jaspers (Marine Biodiscovery Centre: University of Aberdeen)
Oceans cover 70 per cent of planet Earth and it is only in the 20th century that organisms from this environment began to be assessed for their potential to provide new drugs. A new marine derived pharmaceutical pipeline has started flowing, delivering new chemical entities with unique modes of action in treating human disease…
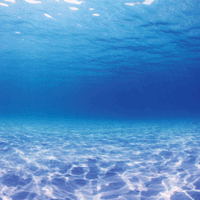
The origin of a drug shouldn’t matter; what is important is its efficacy in treating human disease. Why then do I become excited when another drug derived from a marine source is approved for the clinic? It is the validation of a 60 year-old quest in which a small band of scientists have worked together to find unique molecules from the farthest reaches of life and then develop them into pharmaceuticals, despite the many obstacles. Last year saw the seventh such approval and the pipeline of marine derived molecules in clinical trials contains another 11 candidates at various stages of development, supported by a large pipeline of candidates in preclinical evaluation.
The importance of origin
Why is the origin of these molecules important? Their biological activity certainly merits note – many drugs in the pipeline for pain, cancer and cognitive diseases have unique mechanisms of action. The huge chemical diversity of marine derived molecules is clear and differs from that derived from terrestrial sources, thus making intellectual property protection easier but challenging for synthetic chemists. This chemical diversity is derived from a huge marine biodiversity; the extent of which is still unclear, but is at least in excess of 30 animal phyla compared with 12 for terrestrial habitats. Marine microbial diversity cannot yet be reliably estimated, but is expected to follow a similar trend. The biodiversity is enormous and most species live in habitats that provide stresses which stimulate the production of defensive chemicals with specific and potent activity.
Investigation
The investigation of these biological resources has involved scientists working in many disciplines, and has taken a number of routes; for instance, using ecological studies and broad surveys of the various phyla to discover biological activity. The investigation of cone snail toxins is a relative newcomer to animal toxin studies. These small, beautiful, tropical snails catch a variety of prey that are often fast-moving and bigger than them, and produce powerful venoms that can immobilise and kill their prey almost instantly. The mixture of venoms produced, often called a ‘cabal’, contain a variety of peptidic toxins with different activities. The discovery that some of these were potent painkillers with different mechanisms of action from all other known painkillers excited the neuroscience community. The possibility of new analgesics with fewer side‑effects that were useful for treating different types of pain was a very attractive prospect. The development of Prialt, which is able to treat intractable pain caused by nerve damage – something untreatable by any other type of analgesic – provided a first proof of principle. The compound came from the Sorcerer’s cone snail Conus magus and was developed by Neurex, which was later acquired by Elan (Azur Pharma holds worldwide rights, except for Europe). Its unique activity gained it approval for neuropathic pain via intrathecal administration, and the route is an indication of the possible difficulties associated with the application of peptidic drugs.
Cancer chemotherapeutics
The route to discovery varies from drug to drug, but for cancer chemotherapeutics derived from marine sources, the path to success shows some similarities. Access to broad phylogenetic diversity is always the starting point, followed by extraction and isolation of active principles using a bioassay‑guided isolation protocol. The Caribbean sea squirt Ecteinascidia turbinata was shown by two US groups to have potent cytotoxic activity as a result of a compound called ecteinascidin‑743, which was shown to be effective in animal models. The rights to the compound were acquired by PharmaMar, a Spanish company who used a variety of methods to overcome the major barrier to clinical evaluation, which was access to a reliable supply of material. Initial material was provided using aquaculture techniques, similar to those used for mussels, but this proved to be a very expensive and variable supply route. PharmaMar developed a semisynthetic process starting with safracin‑B, obtainable by microbial fermentation. This process was in excess of 20 synthetic steps, but was more economic than total synthesis. In 2007, the EMEA approved Yondelis (trabectidin, ecteinascidin‑743) for use against soft‑tissue sarcoma. Subsequent trials gained approval for Yondelis as treatment for ovarian cancer and trials for other indications are on-going. PharmaMar is developing a number of other marine‑derived therapeutics in oncology at various stages of development, and is the leader in marine‑sourced cancer therapeutics.
Chemical synthesis
A second example of route to discovery is the use of function-based chemical synthesis to develop a simpler version of halichrondrin B, first derived from the Japanese sponge Halichondria okadai. The initial compound is extremely complex, but has extraordinarily high activity. Natural supply was not an option, and total synthesis, though possible, was not economic. Chemists at Eisai developed a (approximately) 70‑step route to a simpler analogue called Halaven (eribulin), which was approved by the FDA for metastatic breast cancer in 2010. In chemical synthesis, this is a massive achievement, unlikely to be exceeded, and probably represents the limit of feasibility for industrial synthetic chemistry.
What next then for the future development of therapeutics based on molecules derived from marine sources? Most compounds isolated from marine invertebrates are of microbial origin; the realisation of this has led to an explosion of interest in marine micro‑organism‑derived compounds and an understanding of their biosynthesis. A key example is salinisporamide (NPI‑0052), being developed by Nereus Pharmaceuticals for multiple myeloma (Phase I), which is obtained from Salinispora tropica, a marine bacterium. Fermentation to obtain clinical trial‑grade material was done in saline media under contract by IRL Biopharm of New Zealand. Clearly, marine bacteria produce novel compounds with specific and potent activity, and supply via fermentation favours the rapid development of these compounds. As a result, most exploration of marine‑derived pharmaceuticals now focuses on marine micro‑organisms.
Large projects have been launched over the last two years to explore the potential of microorganisms for marine biodiscovery. Three large projects (EUR 8 – 10 million) in particular, funded by the EU’s 7th Framework Programme, are worthy of note: MicroB3 intends to use a combination of sampling and cultivation coupled with a metagenomic and bioinformatics approach to define new targets for biotechnological application. The second project, MaCuMBa aims to develop new isolation and cultivation approaches to discover unique microorganisms with the potential to produce new chemistry. The key to both these projects is developing an understanding of the marine ecosystem to devise approaches that enable the successful cultivation of these strains and eliciting the production of their full biosynthetic potential. The industry-led PharmaSea project puts all the pieces of the biodiscovery pipeline together to discover new chemical entities with potent and selective activities in antimicrobial and central nervous system disease based screens. The source of the microbial diversity for this project is extreme marine environments: deep trenches, cold environments and thermal vents. The guiding philosophy for the project is that unique environments will yield unique biodiversity, which in turn will yield novel chemistry with high bioactivity. The steps taken include: improving access to deep environments using engineering approaches; selective cultivation and genomic approaches to obtain and assess microbial biodiversity; eliciting biosynthetic potential using stress conditions; chemoinformatic approaches to define chemical novelty and help solve chemical structures and finally novel assay systems with the potential to rapidly carry out animal studies and deliver lead molecules to industry partners. These projects form part of the EU’s Blue Growth Strategy, which views marine biotechnology as a major platform for its knowledge-based bioeconomy, and is committed to funding further marine biodiscovery consortia in its Horizon 2020 programme.
In many cases, the compound of interest obtained from an invertebrate cannot be obtained by the cultivation of a microbe. Some research groups have successfully isolated bacterial strains producing such compounds, but generally this is proving to be extraordinarily difficult. The alternative approach is to discover the genes responsible for the biosynthesis of these compounds and use genetic engineering to express them in a surrogate host. This has been possible in a few cases and holds promise as a way forward. The possibilities are then to use genetic engineering to also increase productivity and provide a sustainable supply route. Production can be done by fermentation of the surrogate host, or possibly even by using isolated enzymes in vitro. Finally, this approach brings with it the possibility of altering the structures of complex compounds to explore the bioactivity of analogues more easily than would be possible by chemical synthesis.
This route is in its infancy, but has multiple advantages: it does not require isolation and cultivation of the microorganism; supply is secure; production can be optimised; and analogues are accessible for investigation. Two approaches are mooted; the first is using isolated and possibly engineered enzymes to carry out biotransformations on natural and synthetic substrates to generate diversity not accessible otherwise. This approach can produce multi-milligram quantities of material, which is sufficient for initial discovery and preclinical evaluation in animals. The complementary approach, that of altering the pathway in a microbial host, is effective both for discovery and by using process optimisation, for the large scale sustainable production of any new chemical entities chosen to go forward for clinical trials.
Conclusion
Many disciplines contribute to the successful development of a therapeutic based on a marine‑derived compound, perhaps more than in the standard model of drug discovery. The efficient interplay of all these fields has resulted in the approval of a number of unique therapeutics with novel modes of action. The timeline for developing drugs from marine invertebrates from discovery to the clinic is long, usually because of the lack of a reliable supply. As examples, ecteinascidin‑743 was discovered in 1990 and approved in 2007, and halichondrin‑B was first reported in 1986 and gained clinical approval in 2010. Their exquisite activity and novel modes of action, however, made the effort worthwhile. Using alternative sources such as marine bacteria has speeded up the time to entry into clinical trials – Salinisporamide, for example, went into Phase I clinical trials only three years after it was initially reported, thus showing the value of using microbial sources. Developing new techniques to manipulate biosynthetic pathways will lead to alternative ways to find, produce and modify molecules of marine origin, and thus enable the full potential of marine‑derived therapeutics to be realised.
Related topics
Drug Discovery, Therapeutics
Related organisations
Aberdeen University
Related people
Marcel Jaspers