Academic high-throughput screening; Opportunities for change
Posted: 9 April 2015 | Tim Hammonds (Deputy Director of Discovery: CRT Discovery Laboratories)
Tim Hammonds from Cancer Research Technology Discoveries explains how academic HTS laboratories came into being and outlines the challenges facing the new HTS model…
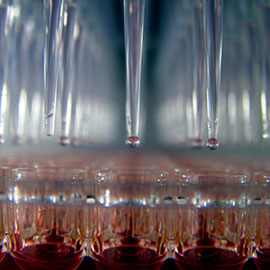
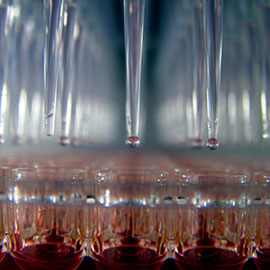
Academic high-throughput screening (HTS) laboratories have evolved from humble beginnings to play a major role in advancing translational research. They now stand at a crossroads where opportunities abound. Big Pharma are dedicated to release more and more of their compound libraries, academics are creating more disease relevant assays and equipment and reagent suppliers are creating more sophisticated hardware and assays at affordable prices. The question facing most groups now is which way to go and why? Focussing on experiences in oncology in the UK, this review looks back at the emergence of academic HTS and looks forward to different, more successful, times ahead.
In describing the evolution of academic HTS laboratories over time, Tim Hammonds from Cancer Research Technology Discoveries explains how academic HTS laboratories came into being. These organisations were often directly funded by universities and institutes. “The new centres were driven by an academic desire to capitalise on the emergence RNA interference technology, which was widely touted as the way to selectively explore cellular mechanisms and find drug targets,” Dr. Hammonds explains. Elsewhere in his article, Hammonds says: “By the mid-late 2000s the scene was set; alongside the expansive Pharma efforts, academia in the US, UK, mainland Europe and Australia had their own exploratory and discovery HTS centres. The strategy was simple: academia and Pharma would reveal target proteins by deep sequencing the genome of diseased cells and/or using siRNA technologies and then each would make a choice as to which of them to prosecute via HTS. Following this, Pharma’s proven capability to make good quality drug-like molecules would eventually create the next wave of targeted agents.” He goes on to outline the challenges facing the new HTS model: namely the global recession and the lack of novel targets emerging from biological studies. In terms of the latter, he draws on some oncology examples to illustrate his point, before going on to discuss phenotypic small-molecule HTS and the potential for the HTS community to exploit “deprioritised molecularly-targeted agents that exist in the compound stores of Pharma and Biotech.”
HTS and targeted drug discovery
From around the mid-1990s, the widespread use of combinatorial chemistry, the sequencing of the genome and the automation and miniaturisation of assays into microplates converged to form a discovery arms race in the pharmaceutical industry. Each company wanted a greater number of compounds in their diverse libraries, with the intention of screening these libraries against the newly emerging molecular targets that would soon be apparent from our increased understanding of how cells work. In this race to develop the next generation of blockbuster drugs, companies invested in million-plus compound libraries, dedicated high throughput and ultra-high throughput screening facilities (the latter presumably for when high throughput is simply not enough) along with all of the associated software and downstream capabilities to interpret and use the data that HTS generates. Screening libraries were described as the ‘crown jewels of Pharma’ and were stored, curated and guarded with seemingly as much pomp and mystery. As HTS expanded, reagent, software and equipment manufacturers made great strides, and indeed great profits, introducing more and more assay formats, kits, hardware, and analysis software onto the market. By the end of the 1990s, high throughput screening had become a science in its own right and was firmly established as the primary way to initiate new drug discovery projects.
The emergence of academic HTS
With HTS seen as the way to start a discovery project, academics wishing to screen their favourite assays found themselves with limited options, the obvious barriers being access to compound libraries and automation hardware. Access to HTS could be obtained via collaborations with the pharma industry stakeholders themselves, albeit with little guarantee of any future involvement in the project, via expensive fee-for-service HTS companies, or indeed by investing in the infrastructure to screen for themselves. The latter was obviously only applicable to the most well-funded of laboratories and lack of funds meant that the capability of academic high-throughput screening groups was a long way behind industry. In the UK in the late 1990s and early 2000s, funding bodies such as the Institute of Cancer Research, Cancer Research UK and the Medical Research Council took it upon themselves to fill this scientific gap. Each built high-throughput screening laboratories at centres dedicated to translational drug discovery. The ICR (Sutton) Laboratories, Cancer Research Technology (CRT) and Medical Research Council Technology (MRCT) were founded with an openly commercially-focussed aspiration, seeking to partner novel discoveries with industry by aligning early-stage drug discovery to the outputs of academic scientists. Compound access was simply overcome by (often shared) purchasing of libraries, typically of tens to hundreds of thousands rather than millions of compounds. However, most equipment manufacturers simply did not cater for small-to-medium-sized HTS facilities, preferring to build room-sized operations for industry. High-throughput (stacker-enabled) plate readers could be purchased in isolation, but liquid handling hardware and HTS analysis software was very difficult and expensive to access. Eventually, thanks in large part to the need for equipment to dispense sequencing reactions and DNA and RNA arrays, medium-scale plate and liquid handling machines became available. By the late 2000s, choosing wisely, an academic HTS facility with an outlay of approximately $1 million on hardware and $1 million on compounds could efficiently equip itself to screen libraries of approximately 100,000 compounds many times a year.
The early days of academic HTS and drug discovery were characterised by an operating model whereby novel, ‘risky’ targets would be prosecuted in collaboration with individual expert academic principal investigators (PIs). By ‘de-risking’ these targets with tractable chemical matter and associated cellular data, the aim was then to partner or to sell assets to a Pharma or biotech partner en route to a novel therapeutic. The point of partnering was typically set at or around in vivo proof-of-concept in animal models and each centre would essentially work at risk on targets until they had a package that was attractive enough to Pharma or biotech.
There then followed a second wave of academic HTS laboratories and these were often directly funded by universities and institutes. The new centres were driven by an academic desire to capitalise on the emergence RNA interference technology, which was widely touted as the way to selectively explore cellular mechanisms and find drug targets. The new academic HTS centres took advantage of the existence of readily available medium-scale HTS hardware and were rapidly set up to prosecute whole genome HTS against cell-based assays. Large-scale cell-based HTS began with relatively simple live/dead readouts, but in order to robustly explore biological systems, academics pushed the screeners to extend the boundaries of which processes can be rapidly and accurately measured in cells. This drive and the emergence of confocal and high resolution digital imaging helped catalyse the emergence of high content screening (HCS), where complex multiparametric readouts are visualised in individual cells across many thousands of wells. So data heavy was HCS when it first emerged that it almost had to wait for Moore’s law to catch up. By the mid-late 2000s the scene was set; alongside the expansive Pharma efforts, academia in the USA, UK, mainland Europe and Australia had their own exploratory and discovery HTS centres. The strategy was simple: academia and Pharma would reveal target proteins by deep sequencing the genome of diseased cells and/or using siRNA technologies and then each would make a choice as to which of them to prosecute via HTS. Following this, Pharma’s proven capability to make good quality drug-like molecules would eventually create the next wave of targeted agents. There seemed to be room for everyone and the perceived advantage shifted from who was capable of performing HTS, to who would find the next blockbuster target to exploit through their HTS.
Problems with the model
Just as the new way seemed so set a high bar, a series of events to a large extent derailed the strategy outlined above. Firstly, the world economy crashed in 2008 and neither the towers of academia nor fortress Pharma were immune from its effects. Programme grants and discovery departments alike were lost to cuts. At the same time, and more importantly, the novel drug targets did not emerge very frequently from the biology studies and the targeted agents that resulted from those that did emerge did not perform as hoped in the clinic. The reasons for this are too detailed for this review of academic HTS, but I use a few oncology examples.
The detailed sequencing of the genome of cancers began to reveal layer upon layer of genetic complexity. This homogenous genetic complexity was not only within an individual’s tumours, but across separate tumours within an individual and even within single primary tumour masses themselves. Results from early siRNA HTS screens have been beset by misinterpretation of false positives, with millions spent chasing these up in multiple Pharma and academic discovery centres. Indeed, the technique is now widely questioned as a drug target discovery technique. Agents such as BRAF inhibitors that specifically target a mutated protein specific to melanoma were successfully brought to market, but their duration of efficacy is limited by the emergence of adaptive pathway related resistance. There have been successes in the clinic, but Glivec, the poster child for durable responses via targeted discovery is beginning to look more and more like the exception to how drugs will be discovered and developed, rather than the rule.
Driven by a lack of success and falling investment in early research, Pharma began to reach out to academia in order to access more, hopefully better, targets. Mechanisms were created that allowed academia to access the crown jewels screening sets and enter into collaborative discovery efforts. Pharma also realised that academic HTS centres were a pivotal gateway to them accessing basic biology and they began to interact with them much earlier in the discovery process. As an example, the 2011 alliance between CRT and Astra Zeneca to discover drugs that target cancer metabolism was formed as a shared risk partnership starting from the target choice stage, a far cry from the early model of waiting until in vivo proof on concept. The landscape had changed dramatically in a few years; there was no longer a feeling of ‘them and us’, but a strengthening realisation that to tackle the complexity of disease and make new drugs it was far more sensible to somehow become ‘us’.
Looking to the future
The fact that the strategy as described has not worked as we had originally hoped is a cause for much disappointment, but rather than abandon concepts, we should learn from our mistakes, look for the silver linings and plan for the future.
In the past 20 years, thousands of targeted small molecule inhibitors have been created by Pharma. These compounds have potent cellular and in some cases in vivo and clinical activity against a significant number of targets spanning many cellular families. There exists an ever expanding smorgasbord of ever cheaper and more high throughput HTS assays and automation technologies. Academia and Pharma continue to push the boundaries of cell-based assays, attempting to design more and more complex, disease relevant systems that work in high throughput, including the use of patient tissues. Alongside these technical advances, Pharma and academia have an increasingly shared vision and are joining forces to form understandings and alliances to explore the best ways to discover new drugs. The question then is which route do we, as academic screeners, take to support this new collaborative model? Moreover what can we do with the assets that are becoming available to us in order to extend the boundaries of drug discovery?
Using cancer as an example and drawing from our experiences at CRT, to my mind there are three key areas where academic HTS can add significant value or lead in the discovery of new therapies; support of the existing target based model, facilitate specialised cellular HCS screens for phenotypic discovery and the repurposing of ‘orphan compounds’, i.e., those deprioritised targeted agents that currently sit idle in the chemical stores of Pharma and academic discovery centres alike.
More of the same: supporting target-based discovery alliances
Where does a small-to-medium academic HTS unit add value to projects in alliances now that the big Pharma screening centres are inside the projects from the outset? Whilst the arrival of a bigger player might seem to be the death knell for a smaller academic screening group, our experience at CRT DL does not bear this out. We have been a part of three early discovery alliances with Pharma companies encompassing 20+ HTS projects over the last four years. We have done all of the HTS for one alliance in house, prosecuted 100K+ subset libraries and performed high-throughput analysis of the hits from partner HTS in the other two. Whilst we have experienced a drop off in full library HTS, having an expert assay and HTS facilities has meant that we have significantly added to alliances in areas where we are equally adept as our larger partners. Assay design and build, hit validation, kinetics analysis and wider compound profiling have featured heavily. Of course, we always leave the very big screens to the enabled partner, but we have found ourselves an equal player in the processes that lead up to and lead from an HTS. HTS has then, against our initial expectations, proven to be a great area of Academia-Pharma collaboration for us and we see no reason for this to change whilst the target based paradigm is prosecuted.
Phenotypic screening: pushing the boundaries.
Following a key publication on the relative success of phenotypic screens versus targeted screens there has been an expansion in phenotypic small molecule HTS and a renewed belief that this is as valid a way to start a discovery programme. Those that previously believed are at the forefront of this and phenotypic HTS consortia and initiatives have been set up around the world. In short, phenotypic HTS has survived the target-based focus, is improving in its scope and size and is here to stay. The advances in HCS and the miniaturisation of standard and three-dimensional cell-based HTS assays has led to the capability to prosecute libraries as large as those previously reserved for biochemical readouts, particularly the 100-200K libraries held in academic HTS centres. There is a huge opportunity to push HCS further and further towards the patient. Complex systems of cell mixtures and/or patient tissues will increasingly be used to build assays and screens for RNA or genetic interference assays, new agents, or to repurpose old agents for new clinical uses. The design and prosecution of more disease-relevant HTS and downstream assays is a key area where academic HTS units previously equipped only with RNAi are uniquely placed; and by this I also mean physically located next to the PIs and Clinicians.
Whilst the emergence of CRISPR/Cas9 technology will no doubt keep the siRNA HTS centres busy for many years to come, these are expert centres for cell based HTS assay design and they should expand those efforts to small molecule screens. By collaborating with Pharma or academic small molecule HTS units they could and should be equipped with good sized small molecule libraries, either as assay-ready nanolitre dispensed plates or acoustic dispense-ready library samples. Proof-of-concept/repurposing screens using these sets will validate the more complex assays ready for potential collaborations to screen larger Pharma sets or provide small molecule starting points for academic studies or discovery projects. This system also works in reverse, whereby collaborations can facilitate cell-based HTS in traditionally biochemical screening units.
Repurposing: focus on preclinical orphan compounds
There are already significant efforts towards repurposing of anticancer agents, but they mostly use individual compounds or sets of marketed agents of a few hundred compounds. The academic HTS community has a perfect opportunity to exploit what I now believe to be the new ‘crown jewels’ of Pharma, namely the deprioritised molecularly targeted agents that exist in the compound stores of Pharma and biotech. The new jewels probably number in the thousands or tens of thousands are not so heavily guarded, most Pharma are willing to offer at least subsets and exemplars for academic studies. If we start now by gathering all of the orphan compounds from all Pharma and Biotech, then the library could be screened by any number of academic centres in non-biased HTS versus the most disease relevant assays that academia can design, with the aim to eventually involve actual patient tissues. By doing this we set a platform for the repurposing of compounds to genetically defined disease subtypes that emerge from the global deep sequencing efforts.
Large funding bodies and charities such as CRUK, NIH, IMI and MRC have a major part to play in this as they are essentially seen as neutral parties by the industrial partners. Hence they can act as a ‘one stop shop’ with single intellectual property agreements to cover all partner interests. They can arrange to gather, curate and store the growing library in a structure blind fashion. The library would be distributed in formats appropriate to each HTS centre and member HTS centres could offer services to those academics that lack HTS. Validated orphan compound hits from repurposing screens then form the basis of discussion and further research as the Pharma guardian is matched to PI on a case-by-case basis. The ultimate goal is the repurposing of the molecule or the discovery of new biology that may be strong enough to reignite an orphaned discovery programme. This is a win-win situation, Pharma may gain a return on the investment in a deprioritised target and academics get access to tool compounds for their biological studies and academic HTS is the key piece in the jigsaw, linking the two together in a way that satisfies both the curiosity of basic research and the rigour of drug discovery research.
Conclusions
We have come a long way in the last 20 years of drug discovery, with many successes in effort, technology build and application but less than spectacular outputs in terms of new small molecule drugs with sustained efficacy. If the target-based paradigm continues to give a low therapeutic yield then we have to accept that change must happen in the way we approach drug discovery. The biology of disease that presents in the patient will always be the same, but our depth of understanding of its complexity will increase. The ways in which we can interrogate that biology, gather, store and process data will inevitably become broader, more efficient, bigger and more powerful. As we learn new techniques, we will have to change the way that we try to discover new hits and ultimately new drugs. Our job as academic HTS units is to continue to work with the best basic biologists and best drug discovery scientists and use our unique bridging position to get the best from both worlds. I think the future is very exciting; as a key part of the larger team there is ample scope to provide quality science to targeted programmes, expand our repertoire of disease-relevant phenotypic HTS and to ultimately explore the best models of disease with an exceptional compound toolkit.
Biography
Tim Hammonds BPharm, PhD, has a degree in Pharmacy and a PhD in pharmaceutical chemistry and microbiology from the University of Nottingham. After five years in enzymology research at the Biochemistry Department at the University of Leicester, Tim joined CRT DL in 1999. During his time there, he has built and ran an assay development, protein production and HTS department. Now Deputy Director of Discovery at Cancer Research Technology Discovery Laboratories, Tim spends his time managing all aspects of translational drug discovery; from the operational aspects of the early drug discovery process, right through to the negotiation and management of multi-site academic/industry drug discovery alliances.
Related topics
Drug Discovery, Oncology, Screening
Related conditions
Cancer
Related organisations
CRT Discovery Laboratories
Related people
Tim Hammonds