Review: Biomarkers towards personalised therapy in cancer
Posted: 2 June 2017 | Katheryn Begg (King's College London), Mahvash Tavassoli (King's College London) | No comments yet
Since the sequence of the human genome was published some 20 years ago, omics strategies have enabled the generation of detailed molecular signatures of cancers and their subtypes.
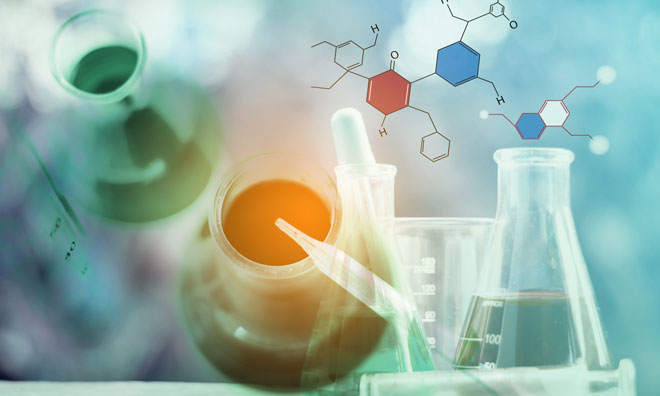
This new information has spurred efforts towards stratification of patients bearing those signatures, development of targeted therapies, and has opened up the paradigm of precision medicine – by using biomarkers. However, the inherent complexity and ever-shifting genetic landscape of cancer has seen many targeted therapies become provocateurs of treatment-induced phenotypes and acquired resistance. Even promising biomarkers that have been identified in attempts to bypass this harbour issues concerning translation and implication into the clinic. Yet there is hope. Evidence from the successful use of biomarkers for diagnosis, screening and management of cancers such as breast and prostate has resulted in earlier diagnoses, higher survival and lower morbidity rates, thus validating the clinical relevance of this concept. Additionally, research into new biomarkers such as PD-1 and CTLA4, and new biomarker classes such as microRNAs and exosomes, coupled with the promise of stronger connections between the realms of molecular biology and biotechnology, means that the new era of biomarker discovery for precision medicine in cancer begins now.
Cancer has existed for millennia, and efforts to fight it have been met with galling failures. We have come a long way from Sidney Farber’s attempts to cure leukaemia by administering folic acid mimics. Following the sequencing of the human genome and development of high-throughput sequencing techniques, the number of genetic signatures illustrative of cancer subtypes has soared. However, in spite of these developments mortality has not significantly improved over the past few decades.1,2 The complex nature of treating a genetically multifarious disease with the propensity to develop therapy resistance quickly is reflected in the low approval rate of new drugs. Furthermore, the ethical and human considerations of sending a drug that may at best marginally improve survival through clinical trials are substantial.
While cancer incidence across our ageing population is increasing, treatment still relies heavily on a technique that has existed as long as medicine itself – surgery – and a cache of chemotherapeutics rife with noxious side effects. The targeted therapies that do exist can push development of further genetic/epigenetic irregularities leading to resistance, metastatic propagation and recurrent disease.3,4 Efficient management of this scenario hangs on identifying suitable treatment strategies from the outset, and monitoring treatment response both throughout and after the course of a disease.
Thus, as the prospect of a cancer cure may seem increasingly distant, development of biomarkers to aid in decision making and predict treatment outcome for personalised therapy now represents the Holy Grail of cancer treatment.
What are biomarkers?
Though many definitions exist, the term biomarker can be applied to any measurable biological entity that is representative of a physiological state.5 They are classed in the following way:
- Diagnostic
- Prognostic – providing a forecast of a patient’s disease progression, irrespective of treatment strategy
- Predictive – allowing insight into likely response or resistance to a targeted therapy.6,7
Omics have represented the major route for biomarker discovery. Most have been identified following delineation of genetic signatures in biopsy tissue. Other omics and deep-sequencing strategies are likely to uncover a wealth of information in relation not only to protein-coding genes, but non-coding elements such as microRNAs, as well as proteins and metabolites.8-13
Biomarkers are detectable either in tumour tissue at the point of biopsy, or circulating in blood, urine and other fluids. Tissue biomarkers are not considered minimally invasive and time to detection and analysis can be long. However, while circulating markers can be easier to monitor during disease progression, their origin and accuracy is often put into question.
Ideally, quantification of biomarkers should also generate reproducible, highly specific and sensitive information. Statistical analysis of biomarker efficacy is a notably important step in development, particularly for the translation from bench to clinic. Interested readers are directed to the reviews by Buyse et al 7 and Duffy et al.14
Despite concerted efforts in biomarker development, only a limited number have been approved for clinical use and so far, no single marker is used independently. Commonly, biomarkers are used in combination with additional features such as tumour grade and differentiation status. The following summarises some of the best studied.
Tissue biomarkers – breast cancer evidence
Between 1998 and 2003 enormous and commendable effort was invested into refining subtypes of cancer, particularly breast cancers (BC).15,16 Similar attempts were made for other cancers,17-22 but none have quite reached the level of clinical significance as in BC. Crucially, this work led to delineation of subtypes characterised exclusively by expression of targetable genes. Table 1 lists biomarkers in the form of genetic rearrangements, fusions and mutations, mostly identified in those pioneering screens. The majority of these markers, as well as having a functional role in oncogenesis, can stratify patients to be treated with therapies designed against them or the pathways through which they function.
Breast cancer consists of numerous subtypes, bearing differences in molecular and phenotypic characteristics.23 The following four major subtypes classify patients:
- Overexpressing or with amplified HER2 = HER2 overexpression
- Negative for HER2 with overexpression of hormone receptors = Luminal A
- Positive for HER2 and hormone receptors = Luminal B
- No overexpression of these markers = Triple negative
A number of other classifiers exist to further group these patients, including the Ki-67 index.24,25
Crucially, alongside the characterisation of BC subtypes has been the development of assays to further predict outcomes including metastasis and local recurrence, and response to therapy. These include MammaPrint, a 70-gene microarray to prevent low-risk patients undergoing unnecessary treatment,26 and Oncotype DX, a 21-gene assay for evaluating recurrence.27,28 These assays act as independent, minimally invasive and accurate biomarkers, and have begun to prove the value of incorporating biotechnological methods in biomarker application.
Circulating tumour markers – prostate cancer evidence
Circulating tumour-associated markers have enhanced diagnostic specificity and prognostication used in conjunction with conventional methods.^29 They are often overexpressed as a by-product of upregulated or amplified oncogenes within the tumour, or released at higher concentrations from an enlarged tissue.30
The introduction of the prostate specific antigen (PSA) as a measure of prostate cancer (PC) has significantly improved PC management, with earlier diagnoses and fewer deaths.31 Use of PSA has also increased the number of cases diagnosed at the localised level leading to fewer deaths from metastases.30,32 This marker demonstrates efficacy as a prognostic indicator in patients, as high levels correlate to clinical stage and Gleason scores.33
Though useful, PSA lacks accuracy as an ideal biomarker. A number of patients are initially diagnosed with low-grade PC. Since quality of life is significantly impaired following treatment, these patients are monitored by continual examinations and PSA measurements in a protocol known as active surveillance.34,35 However, PSA can also be increased by other conditions, inflammation, exercise and metabolism.36,37 Also, paradoxically, very low levels of PSA are occasionally observed in a highly aggressive form of PC.4,38
In an attempt to overcome accuracy issues, the use of derivatives and isoforms of PSA has been suggested. However, there have been conflicting results in their ability to improve specificity whilst maintaining the economic benefits of the PSA test.39 Additional markers for PC include use of the 4K score, the prostate health index, and urine biomarkers such as the TMPRSS2:ERG gene fusion.32,39,40
Breast |
HER2 positive expression, Hormone receptor negative (HER2 overexpression subtype) |
IHC (of full protein or extracellular domain), CISH (for amplification), FISH |
Yes |
Yes |
Yes |
trastuzamab, ado-trastuzumab emtansine, lapatinib, pertuzumab |
Hormone receptor positive, HER2 negative, (Luminal A subtype) |
IHC |
Yes |
Yes |
Yes |
palbociclib, hormonal therapy, eg, tamoxifen |
|
Hormone receptor positive, HER2 positive (Luminal B) |
IHC |
Yes |
Yes |
Yes |
Hormonal therapy, eg, tamoxifen |
|
Hormone receptor and HER2 negative (triple negative) |
IHC |
Yes |
Yes |
olaparib* |
||
Breast, prostate |
Ki-67 |
IHC |
Yes |
Yes |
– |
|
Ovarian |
BRCA1/2 mutation/loss |
IHC |
Yes |
olaparib |
||
CML, ALL |
Philadelphia chromosome-positive BCR-ABL fusion |
Karyotyping, FISH, or qPCR |
Yes |
Yes |
bosutinib and nilotinib (CML), ponatinib, dasatinib, imatinib |
|
CML |
BCR-ABL T315I mutation** |
qPCR |
Yes |
Yes |
Yes |
ponatinib |
GIST |
KIT mutation |
qPCR |
Yes |
imatinib |
||
CML |
||||||
GIST |
PDGFR |
qPCR, FISH |
Yes |
|||
CRC, NSCLC |
KRAS mutations |
IHC, qPCR |
Yes*** |
– |
||
Metastatic melanoma, NSCLC, RCC |
PD-1 overexpression |
IHC |
Yes |
Yes |
nivolumab |
|
Hodgkin lymphoma |
pembrolizumab |
|||||
Urothelial carcinoma, NSCLC |
PD-L1 |
IHC |
Yes |
Yes |
atezolizumab |
|
Advanced melanoma |
CTLA4 |
IHC |
Yes |
Yes |
ipilimumab |
|
NSCLC, HNSCC, (KRAS-wild-type) CRC |
EGFR mutations |
IHC, qPCR |
Yes |
Yes |
gefitinib, erlotinib (NSCLC), cetuximab (HNSCC), panitumumab (CRC) |
|
Melanoma |
BRAF V600 mutation |
qPCR |
Yes |
Yes |
vemurafenib |
|
NSCLC |
ALK rearrangement (EML4-, KIF5B- and TFG-ALK fusions) |
FISH, IHC |
Yes |
Yes |
ceritinib, crizotinib |
Table 1: Current molecular biomarkers used for diagnosis or treatment monitoring in cancer
Immunogenic biomarkers
The diverse genetic reconfigurations that occur during oncogenesis provides the immune system with tumour-associated antigens,41 which are recognised and elicit an immune response. They include the carcinoembryonic antigen (CEA), carbohydrate antigens 15-3, 19-9, 125 and 145, and cancer testis antigens (Table 2). These are upregulated specifically in cancer, or in limited circumstances in healthy people.42
Similar to PSA, these antigens are often overexpressed as a result of aberrant expression or post-translational modifications of genes.43-45 Though they have demonstrated high sensitivities and specificities for diagnosis,45,46 their biology is still uncertain.42,47 As well as utility in diagnosis, they also have potential as prognostic/predictive biomarkers, with evidence of upregulation sometime before clinical presentation and function in promoting oncogenesis and metastasis.48-50 Additionally, their properties as immune-detectable antigens make them potential candidates for immunotherapy or cancer vaccines in the future.
A key recent discovery that certain proteins act as ‘immune checkpoints’ by regulating the T-cell response has led to a deeper understanding of how tumours modulate immunity. These proteins usually function to prevent autoimmunity51 but are also overexpressed in tumours that co-opt these functions as a means of generating an oncogenic immune-privileged environment. They include programmed death protein PD-1, its ligand PD-L1, and cytotoxic T-lymphocyte associated protein CTLA-4.
Immune checkpoint blockade immunotherapies have recently been developed, and their clinical success has been by no means insignificant. Indeed, anti-CTLA-4 monoclonal antibody ipilimumab was the first drug to improve survival for metastatic melanoma patients.51,52 Currently, clinical approval is limited to a few cancers, but considering widespread aberrant expression of PD-L1, PD-1 and CTLA-4, it is likely these drugs will soon be approved for others.53-57
Not all patients who respond to these drugs, however, show a higher expression of immune checkpoints, and vice versa.58 The drugs have also been shown to induce quite severe autoimmune effects.59-61 Thus, further biomarkers are needed to stratify patients, in order to fully harness the potential of this exciting new development.
Oncogenic infectious agents as biomarkers
Risk factors for head and neck squamous cell carcinomas (HNSCC) include high alcohol and tobacco consumption, and infection with human papilloma virus (HPV). HPV acts as a risk factor, oncogenic driver and, due to markedly improved responses to therapy observed HPV-positive patients, it is also an important prognostic biomarker.62
HNSCCs are treated with surgery followed by radiotherapy, which carries significant morbidity. Use of HPV as a biomarker could allow for dose de-escalation during radiotherapy treatment to minimise adverse effects for low-risk patients. Evidence has demonstrated success in this concept,63 but since not all HPV-positive patients will belong solely to the HPV-positive class, there are risks associated with undertreating patients.
In cervical cancers, HPV infection is not as clear a prognostic biomarker, with conflicting results dependent on serotype,64,65 and in contrast to HNSCC, patients generally have a bad prognosis.
Other cancers associated with infection include gastric cancer (GC) patients harbouring Helicobacter pylori infection, and nasopharyngeal carcinoma (NPC) patients with Epstein Barr virus (EBV) infection. H pylori infection is considered necessary for progression to cancer in the majority of GC cases.66 Likewise, EBV infections are found in almost all NPCs. EBV DNA can also be detected in blood, with high levels correlating to advanced disease and poor prognosis.67,68
For each of these cases, not all infected individuals go on to develop cancer. Thus, work is needed to understand why cancer develops in some but not all cases of infection, and to further investigate the biomarker potential.
MicroRNAs as biomarkers
With the view that an ideal biomarker is minimally invasive, robust and accurate, microRNAs (miRNAs) have emerged as ideal candidates. miRNAs have been implicated in almost every cancer and in mechanisms including metastasis,69,70 hypoxia71,72 and resistance to therapy.73-77 miRNAs have the potential to be prognostic/predictive biomarkers, given that miRNA signatures have been shown to change throughout the course of disease and be indicative of crucial cellular processes involved in therapy response.78 A recent study by Tavassoli et al identified miR-196a among miRNAs upregulated in patients who failed radiotherapy, and miR-9 in patients with good response.79 Other studies have identified miR-218 as a tumour-suppressive miRNA modulating chemosensitivity and inhibiting metastasis in a number of different cancers.75,77,80,81 Additionally, they have gained renewed attention as biomarkers given that they are easily detectable and stable in circulation, with levels shown to accurately reflect miRNA levels in the tumour.82,83
Translation of these markers into the clinic has been particularly challenging. One reason being that they have been found to be affected by factors such as age and gender,83 circadian rhythms84 and infections with HPV or H pylori.85,86 Full realisation of their potential must follow from investigations as to the origins of circulating miRNAs and their functions.
miRNAs are not, however, the only species of nucleic acids detectable in blood. Genomic and epigenomic alterations in cell-free circulating DNA from the tumour could represent exciting potential as biomarkers in liquid biopsies,87-90 as well as exosomes91-93 and circulating tumour cells.94
Antigen |
Cancer |
Alpha-fetoprotein |
Liver |
CEA |
Colorectal, breast, lung, liver, pancreatic, stomach and ovarian |
CA 15-3 |
Breast, colorectal |
CA 125 |
Ovarian |
CA 19-9 |
Pancreatic, colorectal, gastrointestinal |
CA 242 |
Pancreatic |
CA 50 |
Gastrointestinal, pancreatic |
CYFRA 21-1 |
Lung |
Lactate dehydrogenase |
Prostate |
PSA |
|
Cancer testis antigens |
Ovarian |
Table 2: Current cancer-associated antigens used in cancer diagnosis
Imaging biomarkers
Imaging, the main method being PET/CT (positron emission tomography computed tomography), is commonly used in the diagnosis and staging of cancer. In PET/CT, the amount of radiotracer taken up by the tissue increases in tumours and concurrently, higher uptake values correspond to poorer prognoses.95
Several recent studies have investigated the potential of linking imaging and expression of existing molecular biomarkers to enhance their prognostic and predictive values, such as in the recent study by He et al, which connected uptake data to response to endocrine therapy and oestrogen receptor expression in a mouse model of BC.96 Or in other studies by radiolabelling targeted therapies, as described in the review by Van Dijk et al with respect to EGFR-targeted imaging by labelling of cetuximab in HNSCC.97
Hypoxia is an established indicator of poor prognosis, due to its association with resistance to chemo- and radiotherapy.98 Hypoxia-specific radiotracers such as Cu-ATSM or F-FMISO could be used to identify more hypoxic tumours,99 which could help to optimise treatment regimens and dosage plans, or to monitor treatment response. It is likely, though, that the combination use of targeted imaging and genetic signatures will be more sensitive in cancer management, such as has recently been suggested by Suh et al and others for assessment of hypoxia.100,101
What next?
It is likely that, whatever their application, new technologies will be necessary to measure biomarkers at the point of diagnosis. While their discovery has been achieved through high-throughput omics methods primarily on cancer tissue, sensitive, cost-effective and quick methods are now needed for efficient biomarker-driven management of cancer in the clinic. Given this situation, an additional challenge for molecular and cell biologists, clinical oncologists and geneticists alike, lies in bridging the gap between their world and that of biotechnology. Some cross-talk has already helped spur successes in the last 20 years. However, better communication is essential in order to make the best use what has already been, and will be, uncovered.
Biographies
Mahvash Tavassoli is Professor of Molecular Oncology, Head of the Department of Molecular Oncology and the Head and Neck Cancer Lead, King’s College London/King’s Health Partners. She obtained her PhD from the University of Sussex, UK. Following several postdoctoral training positions at Fred Hutchinson Cancer Research Centre, USA, Max-Planck Institute, Munich and the Institute of Cancer Research, London, she joined KCL in 1995. She has led a successful research team in the basic and clinical translation aspects of head and neck cancers. Her lab currently focusses on biomarker studies with the aim to develop molecular signatures that enable the prediction of patient response to radio-chemotherapy and targeted therapies.
After obtaining a BSc in Biological Sciences at the University Leeds, during which time she undertook a research project to develop a ‘biosensor’ for detection of drugs in food sources, Katheryn Begg then obtained an MSc in Biomedical Sciences at the University of Bristol, studying Wnt signalling in Zebrafish. Katheryn is now working towards her PhD at King’s College London using omics strategies and bioinformatics to identify biomarkers of treatment response in Head and neck cancer.
References
- Siegel RL, Miller KD, Jemal A. Cancer statistics, 2017. CA: A Cancer Journal for Clinicians. 2017;67(1):7-30.
- Magadi W, Exarchakou A, Rachet B, Coleman MP, Jenkins J, Bannister N, et al. Cancer survival in England: Patients diagnosed between 2010 and 2014 and followed up to 2015. Office for National Statistics. 2016. Available from: www.ons.gov.uk/….
- Sequist LV, Waltman BA, Dias-Santagata D, Digumarthy S, Turke AB, Fidias P, et al. Genotypic and Histological Evolution of Lung Cancers Acquiring Resistance to EGFR Inhibitors. Science Translational Medicine. 2011;3(75):12.
- Roubaud G, Liaw BC, Oh WK, Mulholland DJ. Strategies to avoid treatment-induced lineage crisis in advanced prostate cancer. Nat Rev Clin Oncol. 2016;advance online publication.
- Biomarkers Definitions Working G. Biomarkers and surrogate endpoints: Preferred definitions and conceptual framework. Clinical Pharmacology & Therapeutics. 2001;69(3):89-95.
- Kang H, Kiess A, Chung CH. Emerging biomarkers in head and neck cancer in the era of genomics. Nature Reviews Clinical Oncology. 2015;12(1):11-26.
- Buyse M, Sargent DJ, Grothey A, Matheson A, De Gramont A. Biomarkers and surrogate end points – the challenge of statistical validation. Nature Reviews Clinical Oncology. 2010;7(6):309-17.
- Ma YL, Zhang P, Wang F, Liu WJ, Yang JJ, Qin HL. An Integrated Proteomics and Metabolomics Approach for Defining Oncofetal Biomarkers in the Colorectal Cancer. Annals of Surgery. 2012;255(4):720-30.
- Ma YL, Zhang P, Yang JJ, Liu ZH, Yang Z, Qin HL. Candidate microRNA biomarkers in human colorectal cancer: Systematic review profiling studies and experimental validation. International Journal of Cancer. 2012;130(9):2077-87.
- Lee JW, Lee SY, Lee JE, Park YH. High Resolution Metabolomics to Discover the Potential Biomarkers in EGFR Mutated Lung Cancer. Journal of Thoracic Oncology. 2015;10(9):S582-S.
- Shi HC, Li X, Zhang QY, Yang HM, Zhang XP. Discovery of urine biomarkers for bladder cancer via global metabolomics. Biomarkers. 2016;21(7):578-88.
- Tumas J, Kvederaviciute K, Petrulionis M, Kurlinkus B, Rimkus A, Sakalauskaite G, et al. Metabolomics in pancreatic cancer biomarkers research. Medical Oncology. 2016;33(12):7.
- Yakob M, Fuentes L, Wang MB, Abemayor E, Wong DTW. Salivary biomarkers for detection of oral squamous cell carcinoma – current state and recent advances. Current oral health reports. 2014;1(2):133-41.
- Duffy MJ, Sturgeon CM, Soletormos G, Barak V, Molina R, Hayes DF, et al. Validation of New Cancer Biomarkers: A Position Statement from the European Group on Tumor Markers. Clinical Chemistry. 2015;61(6):809-20.
- Perou CM, Sorlie T, Eisen MB, van de Rijn M, Jeffrey SS, Rees CA, et al. Molecular portraits of human breast tumours. Nature. 2000;406(6797):747-52.
- Sorlie T, Perou CM, Tibshirani R, Aas T, Geisler S, Johnsen H, et al. Gene expression patterns of breast carcinomas distinguish tumor subclasses with clinical implications. Proceedings of the National Academy of Sciences of the United States of America. 2001;98(19):10869-74.
- Alizadeh AA, Eisen MB, Davis RE, Ma C, Lossos IS, Rosenwald A, et al. Distinct types of diffuse large B-cell lymphoma identified by gene expression profiling. Nature. 2000;403(6769):503-11.
- Bhattacharjee A, Richards WG, Staunton J, Li C, Monti S, Vasa P, et al. Classification of human lung carcinomas by mRNA expression profiling reveals distinct adenocarcinoma subclasses. Proceedings of the National Academy of Sciences of the United States of America. 2001;98(24):13790-5.
- Singh D, Febbo PG, Ross K, Jackson DG, Manola J, Ladd C, et al. Gene expression correlates of clinical prostate cancer behavior. Cancer Cell. 2002;1(2):203-9.
- Verhaak RGW, Hoadley KA, Purdom E, Wang V, Qi Y, Wilkerson MD, et al. Integrated Genomic Analysis Identifies Clinically Relevant Subtypes of Glioblastoma Characterized by Abnormalities in PDGFRA, IDH1, EGFR, and NF1. Cancer Cell. 2010;17(1):98-110.
- Golub TR, Slonim DK, Tamayo P, Huard C, Gaasenbeek M, Mesirov JP, et al. Molecular classification of cancer: Class discovery and class prediction by gene expression monitoring. Science. 1999;286(5439):531-7.
- Ramaswamy S, Ross KN, Lander ES, Golub TR. A molecular signature of metastasis in primary solid tumors. Nature Genetics. 2003;33(1):49-54
- Dawson SJ, Rueda OM, Aparicio S, Caldas C. A new genome-driven integrated classification of breast cancer and its implications. Embo Journal. 2013;32(5):617-28.
- Cheang MCU, Chia SK, Voduc D, Gao DX, Leung S, Snider J, et al. Ki67 Index, HER2 Status, and Prognosis of Patients With Luminal B Breast Cancer. Journal of the National Cancer Institute. 2009;101(10):736-50.
- Rastelli F, Crispino S. Factors predictive of response to hormone therapy in breast cancer. Tumori. 2008;94(3):370-83.
- Cardoso F, van’t Veer LJ, Bogaerts J, Slaets L, Viale G, Delaloge S, et al. 70-Gene Signature as an Aid to Treatment Decisions in Early-Stage Breast Cancer. New England Journal of Medicine. 2016;375(8):717-29.
- Sparano JA, Gray RJ, Makower DF, Pritchard KI, Albain KS, Hayes DF, et al. Prospective Validation of a 21-Gene Expression Assay in Breast Cancer. New England Journal of Medicine. 2015;373(21):2005-14.
- Dowsett M, Sestak I, Lopez-Knowles E, Sidhu K, Dunbier AK, Cowens JW, et al. Comparison of PAM50 Risk of Recurrence Score With Oncotype DX and IHC4 for Predicting Risk of Distant Recurrence After Endocrine Therapy. Journal of Clinical Oncology. 2013;31(22):2783-+.
- Shitrit D, Zingerman B, Shitrit ABG, Shlomi D, Kramer MR. Diagnostic value of CYFRA 21-1, CEA, CA 19-9, CA 15-3, and CA 125 assays in pleural effusions: Analysis of 116 cases and review of the literature. Oncologist. 2005;10(7):501-7.
- Catalona WJ, Smith DS, Ratliff TL, Basler JW. Detection of organ-confined prostate-cancer is increased through prostate-specific antigen-based screening. Jama-Journal of the American Medical Association. 1993;270(8):948-54.
- Shariat SF, Semjonow A, Lilja H, Savage C, Vickers AJ, Bjartell A. Tumor markers in prostate cancer I: Blood-based markers. Acta Oncologica. 2011;50:61-75.
- Gaudreau P-O, Stagg J, Soulières D, Saad F. The Present and Future of Biomarkers in Prostate Cancer: Proteomics, Genomics, and Immunology Advancements. Biomarkers in Cancer. 2016;8(Suppl 2):15-33.
- Antenor JAV, Han M, Roehl KA, Nadler RB, Catalona WJ. Relationship between initial prostate specific antigen level and subsequent prostate cancer detection in a longitudinal screening study. Journal of Urology. 2004;172(1):90-3.
- Klotz L, Emberton M. Management of low risk prostate cancer- active surveillance and focal therapy. Nat Rev Clin Oncol. 2014;11(6):324-34.
- Zhao F, Olkhov-Mitsel E, van der Kwast T, Sykes J, Zdravic D, Venkateswaran V, et al. Urinary DNA Methylation Biomarkers for Noninvasive Prediction of Aggressive Disease in Patients with Prostate Cancer on Active Surveillance. The Journal of Urology. 2017;197(2):335-41.
- Larne O, Ostling P, Haflidadottir BS, Hagman Z, Aakula A, Kohonen P, et al. miR-183 in Prostate Cancer Cells Positively Regulates Synthesis and Serum Levels of Prostate-specific Antigen. European Urology. 2015;68(4):581-8.
- Kindermann W, Lehmann V, Herrmann M, Loch T. Influencing of the PSA concentration in serum by physical exercise (especially bicycle riding). Urologe. 2011;50(2):188-+.
- Mahal BA, Aizer AA, Efstathiou JA, Nguyen PL. Association of very low prostate-specific antigen levels with increased cancer-specific death in men with high-grade prostate cancer. Cancer. 2016;122(1):78-83.
- Ferro M, Buonerba C, Terracciano D, Lucarelli G, Cosimato V, Bottero D, et al. Biomarkers in localized prostate cancer. Future Oncology. 2016;12(3):399-411.
- Demichelis F, Fall K, Perner S, Andren O, Schmidt F, Setlur SR, et al. TMPRSS2 : ERG gene fusion associated with lethal prostate cancer in a watchful waiting cohort. Oncogene. 2007;26(31):4596-9.
- Criscitiello C. Tumor-Associated Antigens in Breast Cancer. Breast Care. 2012;7(4):262-6.
- Mirandola L, Cannon MJ, Cobos E, Bernardini G, Jenkins MR, Kast WM, et al. Cancer Testis Antigens: Novel Biomarkers and Targetable Proteins for Ovarian Cancer. International Reviews of Immunology. 2011;30(2-3):127-37.
- Duffy MJ, Evoy D, McDermott EW. CA 15-3: Uses and limitation as a biomarker for breast cancer. Clinica Chimica Acta. 2010;411(23-24):1869-74.
- Byrd JC, Bresalier RS. Mucins and mucin binding proteins in colorectal cancer. Cancer and Metastasis Reviews. 2004;23(1-2):77-99.
- Ni XG, Bai XF, Mao YL, Shao YF, Wu JX, Shan Y, et al. The clinical value of serum CEA, CA19-9, and CA242 in the diagnosis and prognosis of pancreatic cancer. Ejso. 2005;31(2):164-9.
- Cwik G, Wallner G, Skoczylas T, Ciechanski A, Zinkiewicz K. Cancer antigens 19-9 and 125 in the differential diagnosis of pancreatic mass lesions. Archives of Surgery. 2006;141(10):968-73.
- Mare L, Caretti A, Albertini R, Trinchera M. CA19.9 antigen circulating in the serum of colon cancer patients: Where is it from? International Journal of Biochemistry & Cell Biology. 2013;45(4):792-7.
- Liu L, Xiang JF, Chen RF, Fu DL, Hong DF, Hao JH, et al. The clinical utility of CA125/MUC16 in pancreatic cancer: A consensus of diagnostic, prognostic and predictive updates by the Chinese Study Group for Pancreatic Cancer (CSPAC). International Journal of Oncology. 2016;48(3):900-7.
- Wong NK, Easton RL, Panico M, Sutton-Smith M, Morrison JC, Lattanzio FA, et al. Characterization of the oligosaccharides associated with the human ovarian tumor marker CA125. Journal of Biological Chemistry. 2003;278(31):28619-34.
- O’Brien DP, Sandanayake NS, Jenkinson C, Gentry-Maharaj A, Apostolidou S, Fourkala EO, et al. Serum CA19-9 Is Significantly Upregulated up to 2 Years before Diagnosis with Pancreatic Cancer: Implications for Early Disease Detection. Clinical Cancer Research. 2015;21(3):622-31.
- Pardoll DM. The blockade of immune checkpoints in cancer immunotherapy. Nature Reviews Cancer. 2012;12(4):252-64.
- Hodi FS, O’Day SJ, McDermott DF, Weber RW, Sosman JA, Haanen JB, et al. Improved Survival with Ipilimumab in Patients with Metastatic Melanoma. New England Journal of Medicine. 2010;363(8):711-23.
- Lynch TJ, Bondarenko I, Luft A, Serwatowski P, Barlesi F, Chacko R, et al. Ipilimumab in Combination With Paclitaxel and Carboplatin As First-Line Treatment in Stage IIIB/IV Non-Small-Cell Lung Cancer: Results From a Randomized, Double-Blind, Multicenter Phase II Study. Journal of Clinical Oncology. 2012;30(17):2046-54.
- Le DT, Lutz E, Uram JN, Sugar EA, Onners B, Solt S, et al. Evaluation of Ipilimumab in Combination With Allogeneic Pancreatic Tumor Cells Transfected With a GM-CSF Gene in Previously Treated Pancreatic Cancer. Journal of Immunotherapy. 2013;36(7):382-9.
- Slovin SF, Higano CS, Hamid O, Tejwani S, Harzstark A, Alumkal JJ, et al. Ipilimumab alone or in combination with radiotherapy in metastatic castration-resistant prostate cancer: results from an open-label, multicenter phase I/II study. Annals of Oncology. 2013;24(7):1813-21.
- Kwon ED, Drake CG, Scher HI, Fizazi K, Bossi A, van den Eertwegh AJM, et al. Ipilimumab versus placebo after radiotherapy in patients with metastatic castration-resistant prostate cancer that had progressed after docetaxel chemotherapy (CA184-043): a multicentre, randomised, double-blind, phase 3 trial. Lancet Oncology. 2014;15(7):700-12.
- Cocks M, Taheri D, Ball MW, Bezerra SM, Rodriguez MD, Ricardo BFP, et al. Immune-checkpoint status in penile squamous cell carcinoma: a North American cohort. Human Pathology. 2017;59:55-61.
- Patel SP, Kurzrock R. PD-L1 Expression as a Predictive Biomarker in Cancer Immunotherapy. Molecuar Cancer Therapeutics. 2015.
- Brahmer JR, Tykodi SS, Chow LQM, Hwu WJ, Topalian SL, Hwu P, et al. Safety and Activity of Anti-PD-L1 Antibody in Patients with Advanced Cancer. New England Journal of Medicine. 2012;366(26):2455-65.
- Topalian SL, Hodi FS, Brahmer JR, Gettinger SN, Smith DC, McDermott DF, et al. Safety, Activity, and Immune Correlates of Anti-PD-1 Antibody in Cancer. New England Journal of Medicine. 2012;366(26):2443-54.
- Yang JC, Hughes H, Kammula U, Royal R, Sherry RM, Topalian SL, et al. Ipilimumab (anti-CTLA4 antibody) causes regression of metastatic renal cell cancer associated with enteritis and hypophysitis. Journal of Immunotherapy. 2007;30(8):825-30.
- Mallen-St Clair J, Alani M, Wang MB, Srivatsan ES. Human papillomavirus in oropharyngeal cancer: The changing face of a disease. Biochimica Et Biophysica Acta-Reviews on Cancer. 2016;1866(2):141-50.
- Kelly JR, Husain ZA, Burtness B. Treatment de-intensification strategies for head and neck cancer. European Journal of Cancer. 2016;68:125-33.
- Higgins GD, Davy M, Roder D, Uzelin DM, Phillips GE, Burrell CJ. Increased age and mortality associated with cervical carcinomas negative for human papillomavirus rna. Lancet. 1991;338(8772):910-3.
- Burger RA, Monk BJ, Kurosaki T, AntonCulver H, Vasilev SA, Berman ML, et al. Human papillomavirus type 18: Association with poor prognosis in early stage cervical cancer. Journal of the National Cancer Institute. 1996;88(19):1361-8.
- Shiota S, Yamaoka Y. Biomarkers for Helicobacter pylori infection and gastroduodenal diseases. Biomarkers in Medicine. 2014;8(9):1127-37.
- Kang H, Kiess A, Chung CH. Emerging biomarkers in head and neck cancer in the era of genomics. Nat Rev Clin Oncol. 2015;12(1):11-26.
- Fung SYH, Lam JWK, Chan KCA. Clinical utility of circulating Epstein-Barr virus DNA analysis for the management of nasopharyngeal carcinoma. Chinese Clinical Oncology. 2016;5(2):8.
- Tie J, Pan YL, Zhao LN, Wu KC, Liu J, Sun SR, et al. MiR-218 Inhibits Invasion and Metastasis of Gastric Cancer by Targeting the Robo1 Receptor. Plos Genetics. 2010;6(3):11.
- Kogo R, How C, Chaudary N, Bruce J, Shi W, Hill RP, et al. The microRNA-218 similar to Survivin axis regulates migration, invasion, and lymph node metastasis in cervical cancer. Oncotarget. 2015;6(2):1090-100.
- McCormick RI, Blick C, Ragoussis J, Schoedel J, Mole DR, Young AC, et al. miR-210 is a target of hypoxia-inducible factors 1 and 2 in renal cancer, regulates ISCU and correlates with good prognosis. British Journal of Cancer. 2013;108(5):1133-42.
- Mathew LK, Skuli N, Mucaj V, Lee SS, Zinn PO, Sathyan P, et al. miR-218 opposes a critical RTK-HIF pathway in mesenchymal glioblastoma. Proceedings of the National Academy of Sciences of the United States of America. 2014;111(1):291-6.
- Cho WCS. MicroRNAs: Potential biomarkers for cancer diagnosis, prognosis and targets for therapy. International Journal of Biochemistry & Cell Biology. 2010;42(8):1273-81.
- Bertoli G, Cava C, Castiglioni I. MicroRNAs: New Biomarkers for Diagnosis, Prognosis, Therapy Prediction and Therapeutic Tools for Breast Cancer. Theranostics. 2015;5(10):1122-43.
- Xie J, Yu F, Li D, Zhu X, Zhang XP, Lv ZW. MicroRNA-218 regulates cisplatin (DPP) chemosensitivity in non-small cell lung cancer by targeting RUNX2. Tumor Biology. 2016;37(1):1197-204.
- Li Q, Yang ZL, Chen MY, Liu Y. Downregulation of microRNA-196a enhances the sensitivity of non-small cell lung cancer cells to cisplatin treatment. International Journal of Molecular Medicine. 2016;37(4):1067-74.
- Li JR, Ping Z, Ning H. MiR-218 Impairs Tumor Growth and Increases Chemo-Sensitivity to Cisplatin in Cervical Cancer. International Journal of Molecular Sciences. 2012;13(12):16053-64.
- Hummel R, Hussey DJ, Haier J. MicroRNAs: Predictors and modifiers of chemo- and radiotherapy in different tumour types. European Journal of Cancer.46(2):298-311.
- Suh Y-E, Raulf N, Gaeken J, Lawler K, Urbano TG, Bullenkamp J, et al. MicroRNA-196a promotes an oncogenic effect in head and neck cancer cells by suppressing annexin A1 and enhancing radioresistance. International Journal of Cancer. 2015;137(5):1021-34.
- Lin JJ, Wang WY, Xu QQ, Ye JT. MiR-218 increases sensitivity to cisplatin in esophageal cancer cells via targeting survivin expression. Open Medicine. 2016;11(1):31-5.
- Tian H, Hou L, Xiong YM, Huang JX, She YJ, Bi XB, et al. miR-218 suppresses tumor growth and enhances the chemosensitivity of esophageal squamous cell carcinoma to cisplatin. Oncology Reports. 2015;33(2):981-9.
- MacLellan SA, Lawson J, Baik J, Guillaud M, Poh CFY, Garnis C. Differential expression of miRNAs in the serum of patients with high-risk oral lesions. Cancer Medicine. 2012;1(2):268-74.
- Keller A, Meese E. Can circulating miRNAs live up to the promise of being minimal invasive biomarkers in clinical settings? Wiley Interdisciplinary Reviews-Rna. 2016;7(2):148-56.
- Mehta N, Cheng HYM. Micro-Managing the Circadian Clock: The Role of microRNAs in Biological Timekeeping. Journal of Molecular Biology. 2013;425(19):3609-24.
- Martinez I, Gardiner AS, Board KF, Monzon FA, Edwards RP, Khan SA. Human papillomavirus type 16 reduces the expression of microRNA-218 in cervical carcinoma cells. Oncogene. 2008;27(18):2575-82.
- Gao CP, Zhang ZY, Liu WZ, Xiao SD, Gu WQ, Lu H. Reduced MicroRNA-218 Expression Is Associated With High Nuclear Factor kappa B Activation in Gastric Cancer. Cancer. 2010;116(1):41-9.
- Schwarzenbach H, Hoon DSB, Pantel K. Cell-free nucleic acids as biomarkers in cancer patients. Nature Reviews Cancer. 2011;11(6):426-37.
- Herman A, Gruden K, Blejec A, Podpecan V, Motaln H, Rozman P, et al. Analysis of Glioblastoma Patients’ Plasma Revealed the Presence of MicroRNAs with a Prognostic Impact on Survival and Those of Viral Origin. Plos One. 2015;10(5):20.
- Jamal-Hanjani M, Wilson GA, McGranahan N, Birkbak NJ, Watkins TBK, Veeriah S, et al. Tracking the Evolution of Non–Small-Cell Lung Cancer. New England Journal of Medicine. 2017.
- Abbosh C, Birkbak NJ, Wilson GA, Jamal-Hanjani M, Constantin T, Salari R, et al. Phylogenetic ctDNA analysis depicts early stage lung cancer evolution. Nature. 2017;advance online publication.
- Kharmate G, Hosseini-Beheshti E, Caradec J, Chin MY, Guns EST. Epidermal Growth Factor Receptor in Prostate Cancer Derived Exosomes. Plos One. 2016;11(5):14.
- Thind A, Wilson C. Exosomal miRNAs as cancer biomarkers and therapeutic targets. Journal of Extracellular Vesicles. 2016;5:11.
- Martins VR, Dias MS, Hainaut P. Tumor-cell-derived microvesicles as carriers of molecular information in cancer. Current Opinion in Oncology. 2013;25(1):66-75.
- Friedlander TW, Ngo VT, Dong H, Premasekharan G, Weinberg V, Doty S, et al. Detection and Characterization of Invasive Circulating Tumor Cells Derived from Men with Metastatic Castration- Resistant Prostate Cancer. International Journal of Cancer. 2014;134(10):2284-93.
- Groheux D, Giacchetti S, Moretti JL, Porcher R, Espie M, Lehmann-Che J, et al. Correlation of high F-18-FDG uptake to clinical, pathological and biological prognostic factors in breast cancer. European Journal of Nuclear Medicine and Molecular Imaging. 2011;38(3):426-35.
- He SM, Wang MW, Yang ZY, Zhang JP, Zhang YP, Luo JM, et al. Comparison of F-18-FES, F-18-FDG, and F-18-FMISO PET Imaging Probes for Early Prediction and Monitoring of Response to Endocrine Therapy in a Mouse Xenograft Model of ER-Positive Breast Cancer. Plos One. 2016;11(7):12.
- van Dijk LK, Boerman OC, Kaanders J, Bussink J. PET Imaging in Head and Neck Cancer Patients to Monitor Treatment Response: A Future Role for EGFR-Targeted Imaging. Clinical Cancer Research. 2015;21(16):3602-9.
- Busk M, Horsman MR. Relevance of hypoxia in radiation oncology: pathophysiology, tumor biology and implications for treatment. Quarterly Journal of Nuclear Medicine and Molecular Imaging. 2013;57(3):219-34.
- Lopci E, Grassi I, Rubello D, Colletti PM, Cambioli S, Gamboni A, et al. Prognostic Evaluation of Disease Outcome in Solid Tumors Investigated With Cu-64-ATSM PET/CT. Clinical Nuclear Medicine. 2016;41(2):E87-E92.
- Schöder H, Fury M, Lee N, Kraus D. PET Monitoring of Therapy Response in Head and Neck Squamous Cell Carcinoma. Journal of Nuclear Medicine. 2009;50(Suppl 1):74S-88S.
- Suh Y-E, Lawler KJ, Henley-Smith R, Pike L, Leek R, Barrington SF, et al. Association between hypoxic volume and underlying hypoxia-induced gene expression in oropharyngeal squamous cell carcinoma (OPSCC). British Journal of Cancer. 2017.
Related topics
Biomarkers
Related organisations
King's College London, Leeds University