The impact of phenotypic and functional screening in CNS drug discovery
Posted: 9 April 2015 | Christine Williams (UCB), Wolfgang Fecke (UCB)
Wolfgang Fecke and Christine Williams from UCB discuss the industry’s approach to drug discovery with costs escalating and output failing to achieve the corresponding increases in revenue…
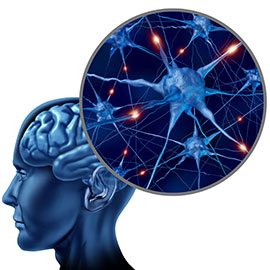
Historically, drug discovery was achieved via the rational design, or serendipitous discovery, of molecules that evoked the desired physiological effects in animals, organs or tissues. This approach is often referred to as ‘classical pharmacology’ or ‘phenotypic’ screening. In contrast, during the post-genomic era and especially during the late-1980s and early-1990s, researchers switched to a more target-based approach using molecular biology to identify targets, create reagents and generate data in in vitro systems employing isolated protein or recombinant cell systems. This approach was appealing to the drug discovery industry as the numbers of molecules that could rapidly be tested was significantly increased and as such a wealth of chemistry and biology techniques were developed to support high-throughput chemistry and assay prosecution, as well as bespoke medicinal chemistry design projects. Such target-based approaches can be successful in identifying new medicines, as illustrated for two drugs in the Central Nervous System (CNS) area, namely aprepitant (Emend®), discovered via high-throughput screening and ramelteon (Rozerem®), designed using conformational restrictions in the endogenous ligand melatonin. However, with costs escalating and output failing to achieve the corresponding increases in revenue, the industry’s approach to drug discovery became highly debated.
An influential article in that respect was that of Swinney & Anthony6 who conducted an analysis of the first-in-class small molecule drugs approved by the FDA between 1999 and 2008. Their analysis indicated that 62% of the drugs approved during that period had been identified via a phenotypic, rather than target-based approach. Interestingly one of the successes cited was memantine, developed more than 50 years ago as an anti-diabetic compound7. This drug was found to have an effect in moderate-to-severe Alzheimer’s Disease8 and is currently being tested in many other CNS diseases (e.g. multiple sclerosis, amyotrophic lateral sclerosis, epilepsy), highlighting that clinical serendipity can play an important role in drug discovery. Indeed recently, Swinney9 has refined his analysis and has indicated that some ‘phenotypic’ approaches can be further divided into those with clinical serendipity, those using in vivo methodologies and those using in vitro cellular systems with a pathway understanding or function marker. On that basis we would highlight that some of their original classifications could be re-defined as functional target-based screens, but none-the-less it is clear that function- or phenotype- based methodologies have played a key role in the discovery of recent drugs. As a consequence there has been a renewed interest in the field of both in vivo and in vitro function-based screening. Whilst the advantages of such approaches can be readily identified, for example early assessment of efficacy, the ability to identify novel pathways and the ability to develop exquisite or beneficial polypharmacology to minimise side effects and maximise efficacy10,11, there are still challenges remaining with this approach. This article highlights some of these challenges and discusses some of the recent progress made, with a focus on CNS disorders, for which Swinney and Anthony6 indicated that 75% of new chemical entities (approved 1999 -2008) were derived via these approaches.
In vivo approaches to CNS screening
When considering some of the recent FDA approvals in the CNS area it is clear that the in vivo route has identified a number of important drugs. For example, the antipsychotic drug aripiprazole (Abilify®) was identified after testing a series of dopamine antagonists in animal models12, aimed at differentiating the pharmacological profile versus other antipsychotic drugs. In addition, the antiepileptic potential of levetiracetam (Keppra®)13 was identified in the 1990s via in vivo means. However, even the success of levetiracetam reflects the difficulties in adopting such an in vivo approach and in particular the challenge in generating models that are valid and predictive of human disease. Specifically, the compound was not effective in acute seizure models such as MES (maximal electroshock seizure) and PTZ (pentylenetetrazole), but was active in models of acquired and genetic epilepsy, in particular in kindling models14. Clearly, many CNS diseases are complex, with multiple (sometimes still unknown) genetic and environmental factors, as well as multiple pharmacologies contributing to the pathology, which makes the development of valid models a challenge.
The translatability of models in CNS has been a key area of investigation over recent years and today there is no consensus on the ‘gold standard’ models to be employed, regardless of the disorder. However, there are many models that are routinely used due to their ability to mimic certain aspects of the human clinical phenotype15. In the case of neuropsychiatric disorders such as depression, anxiety and schizophrenia there are a variety of acute and chronic models that appear to predict for compound effects, at least for those with known mechanism. For example, both acute and chronic models of depression are able to predict for the pharmacological impact of tri-cyclic anti-depressants and selective serotonin reuptake inhibitors16. However, such validity does not always mean the model is mimicking the true complexity of the disease. Despite the success in predicting the pharmacological effects of typical and atypical anti-psychotics, the phencyclidine (PCP) model of schizophrenia does not reflect the negative symptoms of the disease17.
More recently, key emphasis has been placed on the use of genetic and epigenetic information and the development of transgenic models. This is particularly true for the neurodegenerative disorders. However, even for Huntington’s disease, which is caused by a trinucleotide (CAG) repeat in exon 1 of the Huntingtin (HTT) gene, the development of transgenic models is not trivial18. For example, working with the HTT gene is technically difficult and the CAG repeat is unstable in vivo, often leading to a drift and phenotypic differences in generations of the same animal model19. Several rodent models effectively mimic certain aspects of the disease20,21 but none truly reflect the full spectrum of the human condition in terms of motor symptoms, cognitive or behavioural abnormalities. Although a Rhesus monkey model was published several years ago22, none of the animals survived for longer than six months. That said, there is some preliminary evidence that rodent data may predict for human effects, at least in terms of reduced motor effects, when using tetrabenazine (a vesicular monoamine transporter inhibitor)23,24.
In contrast, despite tremendous efforts in the Parkinson’s and Alzheimer’s disease areas, such translatability is yet to be realised. Whilst in Alzheimer’s disease there are now models that develop plaque before tangle pathology, akin to human disease25, only mild cognitive effects are observed at six months and robust pathology and cognitive effects are not observed until the animals are older. Furthermore, disappointing clinical results with vaccination against Ab and application of anti-Ab antibodies mean that questions still remain regarding the validity of these transgenic models26,27. In the case of Parkinson’s Disease (PD), there are a number of autosomal recessive genes that have been identified and knock-out mice developed on this basis, but to date none of them demonstrate the nigrostriatal pathology associated with the disease. Furthermore, whilst the commonly adopted MPTP-treated monkey model demonstrates some dopaminergic loss and a Parkinsonian-like phenotype, other critical characteristics such as Lewy body formation are not present and the translatability of these models, especially for potential neuroprotective therapies, is poor28.
Therefore, it remains clear that whilst in vivo phenotypic screening can be a potentially fruitful way forward, the physiology of such pre-clinical species is inevitably different. Therefore, continued focus on the clinical translatability is key to improving confidence in the results obtained. In the interim, it is not a surprise that many organisations are choosing to adopt more than one model to prevent over interpretation or over-emphasis of the data. Even then, there is no guarantee for the predictive validity of these pre-clinical outcomes.
In vitro approaches to CNS screening
Given the difficulties in generating predictive animal models, many groups are exploring in vitro phenotypic and functional screens as surrogates. Over the course of the last 10 years, several biochemical and cellular Htt aggregation assays have been developed and used for high throughput screening of compound libraries for Huntington’s disease therapies29. For example, neuronal-like PC12 cells were engineered to express intracellular Htt aggregates and used in a screening campaign30. In another example, transcriptional dysregulation caused by the mutated Htt was exploited. A number of hits were identified, some of which were able to rescue cells from mutated Htt-induced cell death31. For PD, a recent high-throughput screen of more than 300,000 compounds identified hits which interfered with the translation of the a-synuclein protein in engineered H4 cells32. The lead compound is currently being evaluated in primary dopaminergic neurons and in animal models for PD.
However, one of the key concerns in developing these in vitro assays is the physiological relevance of such immortalised cell lines. Recently, potent small molecule anticonvulsant agents, with neuroprotective features, have been identified via phenotypic screening of hippocampal cultures with nutrient medium depleted of antioxidants. Cell death and decreased neuronal viability, produced by acute treatment with glutamate or hydrogen peroxide, were prevented by these molecules in this system and activity was confirmed in six animal models33. However, access to such primary cells for CNS disorders can be a challenge. Therefore, many researchers have turned to stem cell systems due to their potential for differentiation into the required cell types. Use of human embryonic stem cells (hESCs) and inducible pluripotent stem cells (iPSCs) to study Huntington’s Disease has been reported by a number of groups and these systems appear to demonstrate some of the phenotypes expected34. In addition, it is believed such an approach is also applicable to a variety of neuropsychiatric indications35. Whilst differentiation protocol optimisation and further characterisation is still required to realise the full potential of stem cells, it is clear that their ability to survive in culture, makes it possible to explore the mechanisms involved in disease in a more relevant cellular setting and temporal fashion. That said, it is also evident that some non-human systems such as yeast may be exploited in some circumstances. Recently, N-Aryl Benzimidazole (NAB) was identified as an active molecule in a screen of about 190,000 compounds, and was found to be more potent against a-synuclein containing yeast compared with yeast which expressed mutated Huntingtin or b-amyloid protein. Although the molecule could not reduce the accumulation of the a-synuclein protein, NAB was shown to be active in a nematode model of PD, a rat primary cortical neuronal model and in human neurons obtained from the differentiation of PD patient-derived induced pluripotent stem cells. As the compound also inhibited growth of wildtype yeast at higher concentrations, this facilitated identification of the molecular target for NAB (namely E3 ubiquitin ligase Rsp5) via screening for yeast mutants which showed no growth inhibition in its presence36. This highlights the potential of such unbiased phenotypic screens to uncover possible therapeutics but also previously unknown biological nodes which may be important in disease pathophysiology.
These examples illustrate the potential of such cellular assays and pathway screens to replace animal models as the primary assay for neurodegenerative disorders. Indeed, to highlight the ultimate impact of such cellular approaches, it is worth noting that the drug perampanel (Fycompa®) was initially discovered via a high throughput screen assessing inhibition of AMPA-induced cortical neuron death37 .Whilst its precise mode of non-competitive inhibition has not been defined, it reached the market in 2012 as an anti-epileptic after its initial exploration as a PD treatment.
Conclusion
It is clear that CNS drug discovery remains a challenging disease area for the industry, but an area with clear unmet medical needs. It is also likely that clinical serendipity will continue to play a part in the identification of novel targets and pathways. For example, rapamycin (Sirolimus®), discovered from a natural product screen as an anti-fungal antibiotic was found to have potent immunosuppressive qualities38. These effects were mediated by binding to a regulatory kinase named mTOR (mammalian target of rapamycin / Sirolimus®) which has subsequently been associated with a variety of genetic diseases39, among them tuberous sclerosis, which often results in intractable epilepsy. Consequently, the mTOR pathway is now seen as a promising new target area for epilepsy40.
However, serendipity alone will not provide for patients needs and as such researchers are placing increasing focus on the development of more and more sophisticated systems to mimic the disease and test compounds. All advances in in vivo understanding and translation will inevitably support drug discovery efforts. However, to facilitate the discovery of the next wave of CNS treatments, it is likely that both a diversity of molecules and pharmacologies need to be screened. As such, the development of in vitro techniques enabling the utilisation of hESCs or iPSCs will be important new tools in the future success of this area. Even for complex disorders such as autism, where it would appear that numerous genes are associated with the pathophysiology underlying the spectrum of the disorder, the outcome of these genetic defects may only affect a limited number of cell types (and synapses) and even fewer neuronal circuits, making functional screening of pathways or relevant phenotypes an effective way forwards.
Acknowledgements
The authors would like to thank Gillian Burgess (UCB) and Henrik Klitgaard (UCB) for their constructive input to the manuscript.
References
- Alvaro, G & Di Fabio, R. Neurokinin 1 receptor antagonists – current prospects. Curr. Opin. Drug Disocov. Dev. (2007) 10, 613-621
- Uchikawa, O et al. Synthesis of a novel series of tricyclic indan derivatives as melatonin receptor agonists. (2002). J
- Munos B. Lessons from 60 years of pharmaceutical innovation. Nat. Rev Drug Discov. 2009, 8:959-968
- Scannell JW, Blanckley A, Boldon H, Warrington B. Diagnosing the decline in pharmaceutical R&D efficiency. Nat Rev Drug Discov. 2012;11(3):191-200.
- Paul SM, Mytelka DS, Dunwiddie CT, Persinger CC, Munos BH, Lindborg SR, Schacht AL.How to improve R&D productivity: the pharmaceutical industry’s grand challenge. Nat Rev Drug Discov. 2010 Mar;9(3):203-14Med. Chem. 45, 4222-4239
- Swinney DC, Anthony J. How were new medicines discovered? Nat Rev Drug Discov. 2011, 10(7):507-19
- Gerzon, K et al. The adamantyl group in medicinal agents. I. Hypoglycemic N-arylsulfonyl-N’-adamantylureas. J Med Chem 1963, 6:760-763.
- Reisberg B et al. Memantine Study Group. (2003) Memantine in moderate-to-severe Alzheimer’s disease. New Engl J Med 2003, 348:1333-41.
- Swinney DC The Contribution of Mechanistic Understanding to Phenotypic Screening for First-in-Class Medicines. J Biomol. Screen. 2013,
- Kotz J Phenotypic screening, take two SciBX 5(15) 1-3
- Zheng W, Thorne N, and McKew JC. Phenotypic screens as a renewed approach for drug discovery. Drug Discov Today. 2013, 18(21-22):1067-1073
- Kikuchi et al. 7-(4-[4-(2,3-Dichlorophenyl)-1-piperazinyl]butyloxy)-3,4-dihydro-2(1H)-quinolinone (OPC-14597), a new putative antipsychotic drug with both presynaptic dopamine autoreceptor agonistic activity and postsynaptic D2 receptor antagonistic activity. J Pharmacol Exp Ther 1995, 274:329-36.
- Klitgaard, H & Verdru, P. Levetiracetam – the first SV2A ligand for the treatment of epilepsy. Expert Opin Drug Discov 2008, 2:1537-1545.
- Klitgaard H, Matagne A, Gobert J, Wülfert E Evidence for a unique profile of levetiracetam in rodent models of seizures and epilepsy Eur J Pharmacol. 1998 24;353(2-3):191-206.
- McGonigle P. Animal models of CNS disorders. Biochem Pharmacol. 2014 1;87(1):140-9.
- Cryan JF, Mombereau C In search of a depressed mouse: utility of models for studying depression-related behavior in genetically modified mice. Mol Psychiatry. 2004 Apr;9(4):326-57.
- Mouri A, Noda Y, Enomoto T, Nabeshima T. Phencyclidine animal models of schizophrenia: approaches from abnormality of glutamatergic neurotransmission and neurodevelopment. Neurochem Int. 2007 51(2-4):173-84.
- Walker, FO. Huntington’s disease. Lancet 2007, 369:218-228.
- Menalled, L et al. Systematic behavioral evaluation of Huntington’s disease transgenic and knock-in mouse models. Neurobiol Dis 2009, 35:319-336.
- Aggarwal, M et al. Spatiotemporal mapping of brain atrophy in mouse models of Huntington’s disease using longitudinal in vivo magnetic resonance imaging. Neuroimage 2012, 60:2086-2095.
- Carroll, JB et al. Natural history of disease in the YAC128 mouse reveals a discrete signature of pathology in Huntinton disease. Neurobiol Dis 2011, 43:257-265.
- Yang, SH et al. Towards a transgenic model of Huntington’s disease in a non-human primate. Nature 2008, 453:921.
- Frank S Tetrabeazine as anti-chorea therapy in Huntington disease: an open label continuiation study. Huntington study group; TETRA-HD Investigators BMC Neurol 2009 9: 62
- Wang H, Chen X, Li Y, Tang T-S, Bezprozvanny I. Tetrabenazine is neuroprotective in Huntington’s Disease mice. Mol Degener 2010 5:18
- Oddo S, Caccamo A, Shepherd JD, Murphy MP, Golde TE, Kayed R, Metherate R, Mattson MP, Akbari Y, LaFerla FM.Triple-transgenic model of Alzheimer’s disease with plaques and tangles: intracellular Abeta and synaptic dysfunction. Neuron. 2003 Jul 31;39(3):409-21.
- Mullane K, Williams M. Alzheimer’s therapeutics: continued clinical failures question the validity of the amyloid hypothesis-but what lies beyond? Biochem Pharmacol. 2013 1;85(3):289-305.
- Sabbagh JJ, Kinney JW, Cummings JL. Animal systems in the development of treatments for Alzheimer’s disease: challenges, methods, and implications. Neurobiol Aging. 2013 34(1):169-83.
- Waldmeier P, Bozyczko-Coyne D, Williams M, Vaught JL. Recent clinical failures in Parkinson’s disease with apoptosis inhibitors underline the need for a paradigm shift in drug discovery for neurodegenerative Biochem Pharmacol. 2006 15;72(10):1197-206.
- Fecke, W et al. Small molecule drug discovery for Huntington’s disease. Drug Disc Today 2009, 14: 453-464.
- Titus, SA et al. High-throughput multiplexed quantitation of protein aggregation and cytotoxicity in a Huntington’s disease model. Curr Chem Genom 2012, 6:79-86.
- Lazzeroni G et al. A phenotypic screening assay for modulators of huntingtin-induced transcriptional dysregulation. J Biomol Screen 2013, 18:984-996.
- Ross, NT et al. Identification of a small molecule that selectively inhibits alpha-synuclein translational expression. In Probe Reports from the NIH Molecular Libraries Program (Internet), 2011.
- Smith GR, Brenneman DE, Zhang Y, Du Y, Reitz AB. Small-Molecule Anticonvulsant Agents with Potent In Vitro Neuroprotection and Favorable Drug-Like Properties. J Mol Neurosci. 2013 Nov 26. [Epub ahead of print]
- Feyeux M, Bourgois-Rocha F, Redfern A, Giles P, Lefort N, Aubert S, Bonnefond C, Bugi A, Ruiz M, Deglon N, Jones L, Peschanski M, Allen ND, Perrier AL. Early transcriptional changes linked to naturally occurring Huntington’s disease mutations in neural derivatives of human embryonic stem cells. Hum Mol Genet. 2012 1;21(17):3883-95.
- K J Brennand, A Simone, N Tran and F H Gage Modeling psychiatric disorders at the cellular and network levels Molecular Psychiatry 2012, 17, 1239-1253
- Tardiff, DF et al. Yeast reveal a “druggable” Rsp5/Nedd4 network that ameliorates α-synuclein toxicity in neurons. Science 2013, 342:979-983.
- Swartz J, Laurenza A. Perampanel. Bialer M, Johannessen SI, Levy RH, Perucca E, Tomson T, White HS, editors. Progress report on new anti-epileptic drugs: A summary of the Tenth Eilat Conference (EILAT X) Epilepsy Res. 2010;92:89–124.
- Martel et al. Inhibition of immune response by rapamycin, a new antifungal antibiotic. Can J Phyiol Pharmacol 1977, 55:48-51.
- Inoki et al. Dysregulation of the TSC-mTOR pathway in human disease. Nature Genet 2005, 37:19-24.
- Ryther, RC & Wong, M. Mammaliuan target of rapamycin (mTOR) inhibition: potential for antiseizure, antiepileptogenic, and epileptostatic therapy. Curr Neurol Neurosci Rep 2012, 12:410-418.
Biographies
Dr Christine Williams is an in vitro pharmacologist with over 17 years of experience in the pharmaceutical industry. She gained her PhD in Biochemistry at the University of Kent with Prof. Philip Strange, where she studied the effects of co-expression of Dopamine receptors with different G-protein alpha subunits. Subsequently, Christine joined Pfizer and then UCB in 2013. During her time in the pharmaceutical industry, Christine has worked in reagent development, high-throughput screening and SAR generating groups. Through this she gained a breadth of scientific, project, portfolio and business management experience across early stage drug discovery. Christine is passionate about the appropriate pharmacological validation of assays and the development of relevant screening cascades for use in drug discovery.
Dr Wolfgang Fecke has worked in the area of pharmaceutical early drug discovery since 1996 and is currently Group Leader in the Primary Pharmacology Department at UCB Pharma (UK). Wolfgang is involved in the identification and characterisation of new NCE and NBE drug candidates in molecular interaction and cellular assays for both immunological and CNS disease targets. Prior to joining UCB, Wolfgang worked for Novartis Pharma and Siena Biotech in the areas of transplant rejection, neurodegeneration and oncology. Wolfgnag obtained his PhD at the Heinrich-Heine-University Dusseldorf in Germany in 1994.
Related topics
Drug Discovery, Screening
Related organisations
UCB Pharma
Related people
Christine Williams, Wolfgang Fecke