Brain organoids: a fascinating and powerful tool for drug discovery
Posted: 5 July 2024 | Dr Francesca Cavallo (Sygnature Discovery) | No comments yet
Human brain organoids are complex in vitro tools derived from stem cells, designed to model the molecular basis of neurodevelopment and the pathogenesis of neurological disorders. By mimicking the function of the human brain, in both health and disease, their application in drug discovery holds significant potential for identifying new treatments for brain disorders, explains Francesca Cavallo from Sygnature Discovery.
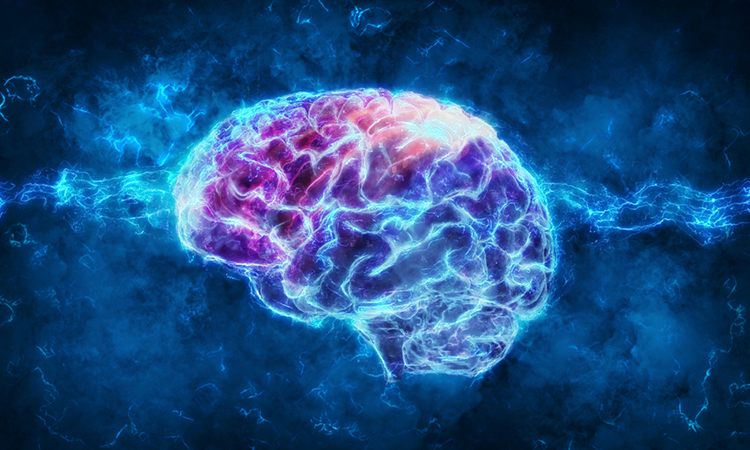
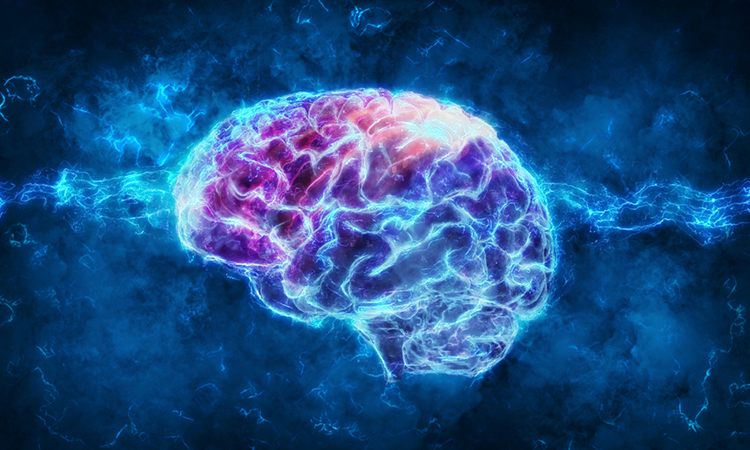
Building three-dimensional models in the lab
Brain organoids are three-dimensional (3D) multicellular structures derived from the differentiation of human pluripotent stem cells (hPSCs), including induced pluripotent stem cells (iPSCs) and embryonic stem cells (ESCs), under specific in vitro neuronal induction conditions, using specific support matrices. They are composed of immature neural progenitors and mature neuronal and glial cells, including astrocytes and oligodendrocytes. The immature cells are located in the inner areas of the organoid, forming ventricle-like structures, and the maturing cells migrate toward the outer areas. This migration results in the formation of multilayered structures.
The high cellular diversity, complex network and self-organised tissue architecture, mean organoids can overcome some of the caveats of two-dimensional (2D) monolayer models, making them a better model of human brain complexity, with the potential to bridge the gap between in vitro 2D systems and in vivo animal models. Further, given their complexity and 3D architecture, they have advantages over 2D systems and are anticipated to better model neurological disorders compared to animal models. Since brain organoids can be derived from either diseased or healthy patients, they can recapitulate human (patho)physiology making them a better choice compared to mammalian cell systems that often fail to recapitulate the human phenotype. Moreover, brain organoids show spontaneous electrical activity, like the human brain, and when transplanted into the mouse spinal cord they innervate the host tissue and establish functional connections with the mouse brain.1
Considerations for creating complex organoids
Despite the numerous advantages of using brain organoids in drug discovery, some limitations should be considered.2 Firstly, they display intrinsic variability in size and morphology, which can arise from both efficiency of neuronal differentiation and heterogeneity in cellular composition. This can be observed between organoids from either the same or different experimental groups. Secondly, organoids lack a functional blood-brain barrier (BBB) and so a drug showing a response in the organoid might not be active in vivo where a BBB is present. However, to overcome this shortcoming, vascularised brain organoids have recently been generated by the fusion of vessel organoids, developed via induction of vascular progenitors, with brain organoids.3 Although these organoids form a complex vasculature, further studies are needed to investigate the presence of blood flow.
Diverse disease applications of brain organoids
Because organoids recapitulate the different stages of human neurodevelopment and can be maintained in culture for up to one year, they hold great promise in studying neurodevelopmental disorders, such as autism spectrum disorder (ASD). ASD is genetically highly heterogeneous, caused by mutations in genes, eg, SHANK3, ANKRD11 and CHD8, which are associated with chromatin remodelling, epigenetic changes, synapse physiology and migration of neuronal cells.4
A strictly related condition that can benefit from these models is Timothy syndrome (TS), a severe multisystem disorder characterised by autism and neuropsychiatric features, caused by gain-of-function mutations in the CACNA1C gene. TS has been studied through the generation of “assembloids”, glutamatergic and GABAergic organoids fused together. This has revealed an alteration in the migration of cortical interneurons, a finding that would be difficult to observe in 2D models. Interneuron migration is regulated by L-type calcium channels (LTCCs), encoded by the CACNA1C gene. Mutations in the CACNA1C gene lead to dysregulation of migration and saltation frequency in interneurons. TS migratory phenotype in assembloids can be reversed by the LTCCs blocker nimodipine, resulting in a reduction in saltation length and speed.5,6
Brain organoids have also been used to investigate stage-specific effects of multiple sclerosis (MS), an inflammatory neurological condition affecting the central nervous system.7 The complex aetiology of MS is driven by both polygenic and environmental factors, thus making it a challenging disease to model in vivo. A hallmark of MS is that the demyelinated lesions cannot be quickly repaired, resulting in inefficient remyelination and axons open to attack by the immune system. The generation of MS-derived oligocortical 3D models containing oligodendrocytes and compact myelin after 30 weeks in culture, represents an attractive platform for preclinical drug testing, as they recapitulate many aspects of MS pathology.8 Oligocortical 3D models have been used to validate the effect of two promyelinating drugs, benztropine and miconazole. Both drugs increased the percentage of mature myelinating oligodendrocytes compared to control groups.9
Recently, a protocol that generates brain organoids containing mature myelinating oligodendrocytes in just 42 days has been established, representing an attractive platform for drug screening, as it has markedly reduced the experimental time course compared to the 30-week model.10 However, cerebral organoids do not contain immune cells, eg, microglial cells, thus inadequately modelling the inflammatory component associated with demyelination in MS.
Integrating microfluidics to brain organoids for optimisation
Although most brain organoids lack immune cells, do not demonstrate blood flow, and have variability in organoid size and cell composition, some of these shortcomings can be overcome by microfluidic technology. Microfluid platforms promote the precise spatial and temporal distribution of non-neuronal cells within the organoids, building a more physiological 3D microenvironment. This technology can also potentially control organoid size through physical constraints that prevent size variations. Furthermore, microfluidics facilitates the generation of vascularised brain organoids composed of perfusable lumens that allow drugs, immune cells and nutrients to access the organoid’s core.11 Incorporating microfluidic biosensors into organ-on-a-chip (OOC) platforms represents an innovative strategy to investigate microenvironment dynamics in brain tumours, such as glioblastoma. Glioblastoma multiforme (GBM) is the most aggressive primary brain tumour in adults, characterised by high malignancy and aberrant vascularisation. The biosensor-OOC micro-engineered models can help elucidate the complexity of GBM by recapitulating brain tumour vasculature and replicating aspects of the tumour microenvironment, comprised of cell proliferation, migration and tangled cell interactions between tumour and endothelial cells.12
Incorporating brain organoids into drug discovery platforms
Ultimately, brain organoids represent a reliable model for drug screening purposes. Recently, a network-based drug-screening platform has been developed to test FDA-approved drugs for Alzheimer’s disease (AD) using 1,300 iPSC-derived organoids from 11 sporadic AD patients.13 Another application of brain organoids is for neurotoxicity studies, by assessing the effect of potential toxicants on neuronal differentiation and network formation during neurodevelopment.
Brain organoids can also be used to evaluate and confirm the efficacy of candidate drugs, previously tested in 2D models, and are an ethical alternative to animal models. A significant challenge for the field is incorporating 3D models into high-throughput platforms.14 However, pharma, biotech companies and contract research organisations (CROs) are actively exploring the potential to scale up organoid screening capabilities (Table 1). Companies and researchers focused on utilising organoids, and more generally humanised assay platforms utilising iPSCs, are increasingly looking to partner with specialist CROs who are able to provide highly experienced teams, technology platforms, and scale to deliver effective solutions to many of the highlighted challenges in incorporating 3D brain organoids into neuroscience research.


Table 1. Companies using organoids and focused on cross-therapeutic areas.
References
- Giandomenico SL, et al. Cerebral organoids at the air-liquid interface generate diverse nerve tracts with functional output. Nature Neuroscience. 22:669-679 (2019). https://www.nature.com/articles/s41593-019-0350-2
- Giorgi C, et al. Brain Organoids: A Game-Changer for Drug Testing. Pharmaceutics. 16(4):443 (2024). https://www.mdpi.com/1999-4923/16/4/443
- Sun XY, et al. Generation of vascularized brain organoids to study neurovascular interactions. eLife. eLife 11:e76707 (2022). https://elifesciences.org/articles/76707
- Chan WK. Cerebral organoids as tools to identify the developmental roots of autism. Molecular Autism. 11 (2020). https://molecularautism.biomedcentral.com/articles/10.1186/s13229-020-00360-3
- Birey F, et al. Assembly of functionally integrated human forebrain spheroids. Nature. 545:54-59 (2017). https://www.nature.com/articles/nature22330
- Villa C, et al. Patient-Derived Induced Pluripotent Stem Cells (iPSCs) and Cerebral Organoids for Drug Screening and Development in Autism Spectrum Disorder: Opportunities and Challenges. Pharmaceutics. 13(2):280 (2021). https://www.mdpi.com/1999-4923/13/2/280
- Daviaud N, et al. Cerebral organoids in primary progressive multiple sclerosis reveal stem cell and oligodendrocyte differentiation defect. Biol Open. 12(3) (2023). https://www.ncbi.nlm.nih.gov/pmc/articles/PMC10040243/
- Madhavan M, et al. Induction of myelinating oligodendrocytes in human cortical spheroids. Nat Methods. 15(9):700-706 (2018) https://pubmed.ncbi.nlm.nih.gov/30046099/
- Deshmukh V, et al. A regenerative approach to the treatment of multiple sclerosis. Nature. 502:327-332 (2013). https://www.nature.com/articles/nature12647
- Shaker MR, et al. Rapid and Efficient Generation of Myelinating Human Oligodendrocytes in Organoids. Frontiers. 15 (2021). https://www.frontiersin.org/journals/cellular-neuroscience/articles/10.3389/fncel.2021.631548/full
- Tan SJ, et al. Vascularized human brain organoid on-chip. Lab on a Chip. 12 (2023). https://pubs.rsc.org/en/content/articlelanding/2023/lc/d2lc01109c
- Thenuwara G, et al. Advances in Diagnostic Tools and Therapeutic Approaches for Gliomas: A Comprehensive Review. MDPI. 23(24):9842 (2023). https://www.mdpi.com/1424-8220/23/24/9842
- Park JC., et al. A logical network-based drug-screening platform for Alzheimer’s disease representing pathological features of human brain organoids. Nature Communications. 12 (2021). https://www.nature.com/articles/s41467-020-20440-5
- Marangon D, et al. Novel in vitro Experimental Approaches to Study Myelination and Remyelination in the Central Nervous System. Frontiers. 15 (2021). https://www.frontiersin.org/articles/10.3389/fncel.2021.748849
About the author
Francesca Cavallo, Senior Scientist at Sygnature Discovery
Related topics
Drug Discovery, In Vitro, Induced Pluripotent Stem Cells (iPSCs), Microfluidic Technology, Neurosciences, Organoids
Related conditions
Alzheimer's disease (AD), Multiple Sclerosis (MS), Neurological disorders
Related organisations
Sygnature Discovery
Related people
Dr Francesca Cavallo (Sygnature Discovery)