Key considerations before commencing cell line development
Posted: 5 July 2023 | Dr Alison Porter | No comments yet
Cell line development (CLD) is a critical, early step when entering the development phase of a biotherapeutic. Access to specialist skills and experience, either in-house or remotely, and to multiple technologies and equipment can prove invaluable for the timely and successful completion of this stage. Here, Dr Alison Porter addresses the first points to consider for in-house CLD and which factors can influence them.
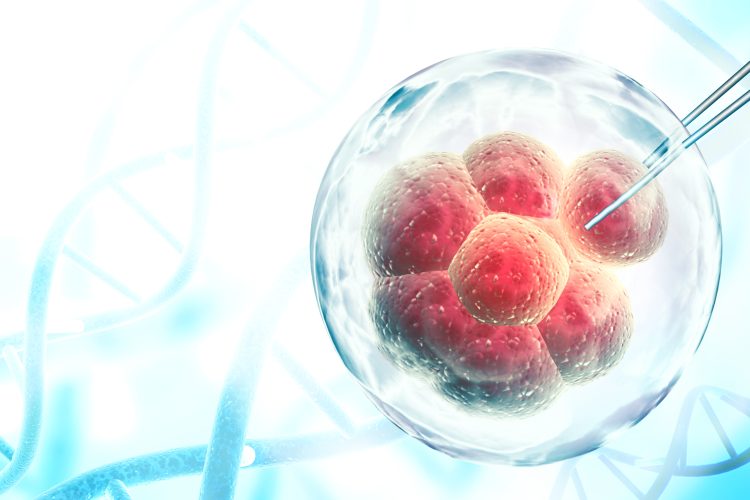
The cell line development stage
The cell line development (CLD) stage begins with gene optimisation for high expression, followed by insertion of the genes of interest (GOIs) into a mammalian host cell line. Typically, this will be a Chinese hamster ovary (CHO) cell line, with alternatives such as murine, human and even duck embryonic cell lines having been used. Although all originate from the one hamster, several different lineages of CHO cell line are available with most being traced back to a CHO-K1, CHO DG44 or CHO-S parent.
A good introduction to the use of CHO cells for biotherapeutics production can be found in an article commemorating the 20th anniversary of the approval of a product produced using CHO cells.1
Another critical component in this first step is the selectable marker that will be inserted into the genome along with the GOIs. This selectable marker enables the identification of cells which have taken up the GOIs and aids the initial selection of recombinant protein expressing cell lines. The marker is typically a metabolic marker (eg, GS or DHFR have been routinely used for many years) but could also be an antibiotic resistance marker. However, use of antibiotic resistance markers may be considered less popular and they certainly are not used once initial transfectants are isolated. This is due to concerns around contribution to antibiotic resistance in the environment.
Once the GOIs have been integrated, a CLD process undergoes several critical steps (see Figure 1) including cloning, expansion of cells, screening and selection. Once completed, and a lead cell line has been identified, a project is then ready to move into other stages such as GMP cell banking and upstream process development.
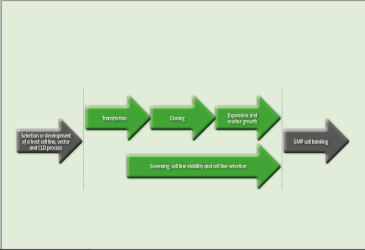
Figure 1: Critical steps in a CLD process
Many companies offer CLD as a service and can complete this stage, among others, for clients. However, some groups will decide to complete this stage within their own laboratories. If taking this approach, there are two critical decisions to be made before work can start:
- What host cell line, vector and CLD process will be used
- What equipment will be purchased.
Host cell line, vector and cell line development process – to outsource or not?
The first critical decision is whether to access one of the commercially available host cell line, vector and CLD platforms on the market, or to develop a novel, proprietary system in house. Team members will consider this from different angles, focusing on factors such as costs (upfront costs, future royalties, etc); freedom to operate; speed to clinic; track record; technology capability and ease of use.
Many commercially available systems provide all three components, and the company may also provide technical support from scientists that specialise in CLD. This benefits the end user and enables increased speed to clinic. Such systems should be ready to go, allowing CLD activities for a chosen molecule to quickly begin. They can also offer the benefit of being known to regulatory authorities, thus increasing the chance of a first-time, successful regulatory review.
We talk about personalised medicine; how about personalised host cell lines?
An additional advantage with some providers is that they may offer access to a portfolio of additional components. A good example here is the potential to access transposon-based technologies such as GS PiggyBac (Lonza) or Leap-In (Atum). Recent years have seen several companies introduce these technologies into their CLD workflows. They work in the following way: the genes in an expression vector are flanked by inverted terminal repeat sequences (ITRs) to which a specific transposase enzyme can bind. After binding, the transposase enzyme will excise the flanked section of DNA (termed the transposon) and insert it into sites in the genome at specific target sequences. These sites are typically in areas of open chromatin and associated with highly expressed genes. Use of such technologies has the potential to improve product concentration, which can be especially useful for more complex biologics that can be harder to express, and speed to clinic. Insertion into these target sites may also improve cell line stability.2,3,4
On the flip side, disadvantages with utilising commercially available systems can include costs and possible restrictions to application. It could therefore be argued that open source platforms to aid the development of new biopharmaceuticals are needed, although this is not without risk as such platforms will, for example, initially be unknown to regulatory authorities.
If in-house development of a novel, proprietary system (host cell line, vector and CLD process) is chosen instead, this holds the obvious advantages of fewer restrictions to application, and developing it for their specific requirements.
However, many of the technologies a developer may wish to include are still proprietary or subject to a third-party patent. An awareness of this is essential: groups should also check for restrictions on commercial use from the source of their CHO cell line. It should be noted that what may have been permissible for an academic will be viewed differently for a commercial entity which will be scrutinised for infringement. Over time, there may also be a cost benefit with developing a proprietary system if there is a pipeline of multiple products to produce.
On the downside, the amount of resource and time needed to develop a successful system should never be underestimated: it entails far more than simply selecting a colony. Taking the host cell line as an example: as a minimum, it will likely need to be adapted to desired culture conditions (animal component-free medium and suspension culture). There will also likely be a need to utilise a directed evolution approach and/or cell line engineering to enhance the desired growth and productivity characteristics, and stages such as cell banking, adventitious agent testing and cell line history preparation can be required. Currently, it can take months to years to establish a high performing host cell line. Further and substantial time can also, of course, be required to develop vectors and the CLD process.
A potential future for host cell lines
There have been many recent advances in cell line engineering technologies and our understanding of CHO cells. An example of the former includes a report in the journal Nature of a new tool that can increase the amount of DNA that can be inserted using CRISPR-Cas9 technology.5 And for the latter, the development of a technique to temporally track gene expression in CHO cells, which therefore has the potential to lead to the identification of targets to improve recombinant protein production.6
As a result, we may well see more options when it comes to host cell lines offered by companies in the future. With the increase in more complex biologics, this could aid their expression (product concentration and product characteristics) if this is problematic. Equally, it may become easier for groups to engineer a cell line to fit their molecule’s particular requirements in their own laboratory. We talk about personalised medicine; how about personalised host cell lines?
Equipment
The second critical decision to make involves which equipment to purchase. This will be closely linked to the design of the CLD process and which methods it will entail. Even the purchase of seemingly simple equipment such as incubators must be carefully considered: will they adequately support all the different vessel types to be used in the process (static multi-well plates, shaken deep-well plates, TPP® TubeSpins, shake-flasks – to name a few potential vessels)? Are additional components needed for an incubator to support a particular vessel type?
The equipment selected can impact factors such as speed to clinic and ability to identify the best cell line.
For example, if working with shaken deep-well plates, a clamping system may be required to hold the plates in place in an incubator along with a lid system to ensure physical conditions (evaporation, gas exchange, etc) are the same across all wells.7,8 This leads into decisions around the design of the process to screen and select the best cell line(s): further equipment may be desirable for improved prediction of cell line behaviour in the production process. For example, a microbioreactor system can be beneficial. When designing the CLD process, simple static multi-well plate and shake flask systems can be used but it should be recognised that simple screens are often weak at identifying the best cell lines due to their environmental differences compared to the bioreactors in which the cell line will eventually be used.9 Ergo, more complex screens that include the use of feeds, suspension culture and environmental controls (such as microbioreactors) are far better at identifying the best cell lines.
Another key point for which equipment will be required is cloning. It currently remains a regulatory requirement for a recombinant protein-producing cell line to be derived from a single progenitor cell (ICH Q5D). However, in more recent years the expectations around how to fulfil this requirement have changed.
Originally, limiting dilution cloning was the method of choice for cloning, although there was a need to perform several rounds of this to achieve a suitably high probability of monoclonality. Due to a desire to reduce timelines by eliminating the need for multiple rounds of cloning, many groups moved away from using limiting dilution cloning and turned instead to using equipment such as a FACS machine for this stage. Although approaches such as limiting dilution cloning and FACS can and are still used, there is now a strong expectation that the cloning process will include imaging as supporting evidence that a cell line originated from a single cell. As such, a plate imaging system will be required from an equipment point of view as a minimum. It is also important to note here that work to demonstrate that the imager is capable of its task will be required.10
In more recent years, several pieces of equipment have become available that combine both the cloning and imaging, to perform the stage in a single round. These devices can save time and labour. Broadly speaking, the equipment can be split into two categories based on their function and cost:
- Devices used purely for cloning (eg, Solentim’s VIPS™ and Molecular Device’s / Cytena’s Single-Cell Printer™)
- Microfluidic devices which combine the ability to perform cloning and cell line screening steps (eg, Berkley Lights’ Beacon® / Beacon Select™ and Sphere Fluidics’ Cyto-Mine®).
Finally, if multiple projects are to be undertaken and there is a need for high throughput, the introduction of integrated liquid handling / automation platforms should be considered.
Summary
Once a group has decided to undertake the CLD stage in their own laboratory, there are two critical follow-on decisions to make, which are not simply and solely influenced by the technology itself:
- What host cell line, vector and CLD process to use
- What equipment to purchase.
From the discussion on whether to access an external company’s host cell line, vector and CLD platform technology or for a group to develop its own, it is evident that numerous factors must be considered. These include time, costs, experience, freedom to do what you want with the system, etc. This decision may be further complicated in future with the potential for increased levels of options for engineered host cell lines.
When it comes to equipment, it is evident that there are options for different budgets. However, what is selected can impact factors such as speed to clinic and ability to identify the best cell line.
Dr Alison Porter
Alison has a PhD from the University of Manchester and over 25 years of experience in mammalian cell culture. She spent most of this time working in the biopharma industry, with companies such as Lonza and Fujifilm Diosynth Biotechnologies. She has extensive experience in multiple areas of bioprocessing, with a specialisation in cell line development for the manufacture of recombinant proteins. Most recently, Alison has applied her biopharma skills in the field of cultivated meat.
References:
- Jayapal KP, Wlaschin KF, et al. Recombinant protein therapeutics from CHO cells — 20 years and counting. Eng. Prog. (2007), 103(7): 40–47.
- Rajendra Y, Peery RB, Barnard GC. Generation of stable Chinese hamster ovary pools yielding antibody titers of up to 7.6 g/L using the piggyBac transposon system. Prog. (2016), 32: 1301-1307
- Matasci M, Baldo L, et al. The PiggyBac transposon enhances the frequency of CHO stable cell line generation and yields recombinant lines with superior productivity and stability. Bioeng. (2011), 108: 2141-2150
- Schmieder V, Fieder J, et al. Towards maximum acceleration of monoclonal antibody development: Leveraging transposase-mediated cell line generation to enable GMP manufacturing within 3 months using a stable pool. Biotechnol. (2022), 349: 53-64.
- Yarnall MTN, Ioannidi EI, et al. Drag-and-drop genome insertion of large sequences without double-strand DNA cleavage using CRISPR-directed integrases. Nat Biotechnol (2022).
- Papež M, Jiménez Lancho V, et al. SLAM-seq reveals early transcriptomic response mechanisms upon glutamine deprivation in Chinese hamster ovary cells. Bioeng. (2023), 1– 17
- Duetz WA, Ruedi L, et al. Methods for Intense aeration, growth, storage, and replication of bacterial strains in microtiter plates. Environ. Microbiol (2000) 66(6): 2641-2646.
- Wang B, Albanetti T, et al. High-throughput screening of antibody-expressing CHO clones using an automated shaken deep-well system. Biotechnol Prog. (2018) 34(6): 1460-1471.
- Porter AJ, Racher AJ, et al. Strategies for selecting recombinant CHO cell lines for cGMP manufacturing: Improving the efficiency of cell line generation. Biotechnol Progress (2010), 26: 1455-1464.
- Zingaro K, Shaw D, et al. Implementation of plate imaging for demonstration of monoclonality in biologics manufacturing development. PDA J Pharm Sci Technol (2018), 72(4): 438-450.
Related topics
Biotherapeutics, Cell Cultures, Cell Line Development, DNA, Genomics