T-cell receptors offer window to the cell for a new class of cancer therapeutics
Posted: 15 January 2024 | Daniel Kavanagh (WCG) | No comments yet
Dr Daniel Kavanagh, Senior Scientific Advisor, Gene Therapies, Vaccines and Biologics at WCG, discusses how monoclonal antibodies are limited by their ability to only recognise tumour antigens expressed on the outside surface of a cell. In this article he explains how investigational genetically engineered medicinal products are leveraging T-cell receptors to allow the immune system to monitor events inside cells, greatly expanding potential immune targets for treating cancer and other diseases.
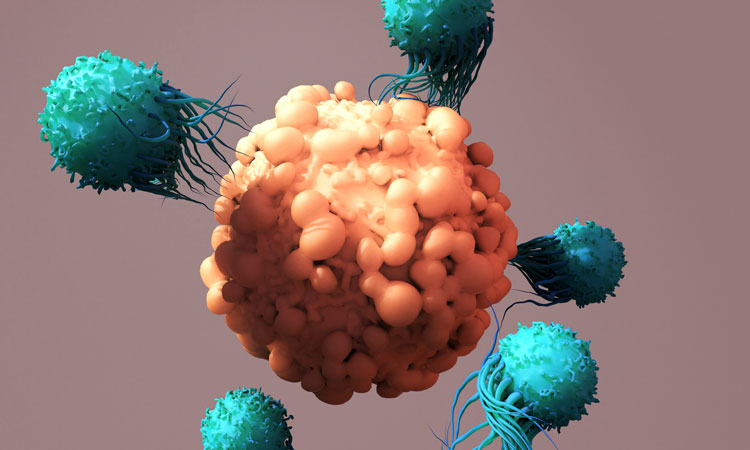
Monoclonal antibodies (mAbs) targeting tumour-specific antigens play an essential role in the treatment of many cancers. In general, these agents work by binding to a molecular target on the surface of a tumour cell, and directing destruction of the target cell by chemical, radiological or immunological mechanisms. This approach has produced a broad range of approved and investigational products. Notable recent examples include CD19‑directed chimeric antigen receptor (CAR) T cells. CD19 CAR T cells are effective because the CD19 antigen is expressed on the surface of many B cell‑derived tumours.
An important limitation of antibodies against tumour antigens is that these agents direct responses to molecular targets present on the surface of the cell. Antibodies in general cannot cross the plasma membrane or direct responses against intracellular targets, whereas many critical steps in oncogenesis and tumour pathology occur inside the cell, concealed from the protective effects of antibodies.
Gaining access inside cells
Fortunately, evolution has provided a mechanism, found in all higher vertebrates, for the immune system to monitor intracellular events. This mechanism relies on proteins encoded by a group of genes called the Class I major histocompatibility complex (MHC); in humans these proteins are also known as class I human lymphocyte antigen (HLA) proteins. Class I HLA proteins provide an elegant mechanism for the display of short peptides, derived from fragments of intracellular proteins, on the surface of the cell (Figure 1). The molecular complex of HLA plus an antigenic peptide is sometimes referred to as “pHLA”.
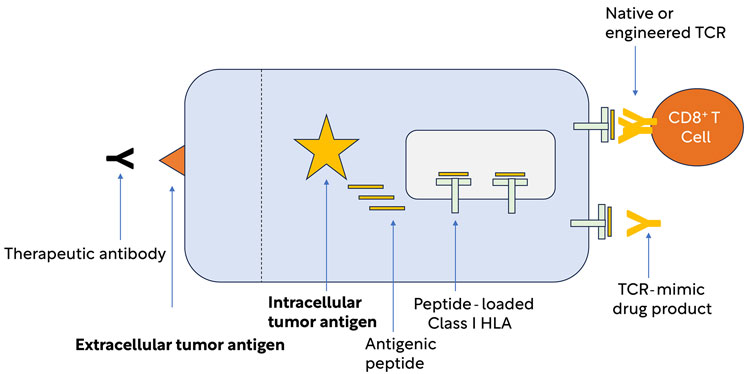
Figure 1: HLA Class I presentation of peptides derived from intracellular antigens. Unlike tumour antigens expressed on the cell surface, intracellular tumour antigens are generally inaccessible to therapeutic antibodies. The Class I HLA system presents fragments of intracellular antigens to CD8 T cells and allows the immune system to monitor intracellular events, thus providing an essential component of immunity against cancer.
CD8+ T cells are a type of lymphocyte that specialises in scanning information presented by HLA Class I. The molecular decoder used by T cells to detect signals in the context of HLA molecules is called the T-cell receptor (TCR). Each T cell expresses a TCR specific for an HLA‑presented peptide signal, and when that signal is detected a T cell expressing the relevant TCR can launch a complex array of immune effector responses that can suppress or destroy tumours or virus-infected cells.
The gene family encoding HLA molecules has many highly specialised features to ensure that T cells can respond to a broad range of rapidly evolving threats. These features include the presence of multiple distinct HLA genes at nearby molecular addresses (loci) on human chromosome 6. There are three Class I HLA loci, designated A, B and C; therefore, geneticists say that this gene family is polygenic. In addition, for each HLA gene, there is no single dominant genetic form. Among the human population there are many prevalent alternative forms, or alleles, of each HLA gene – geneticists say that each HLA gene is polymorphic. As each individual inherits one copy of each Class I HLA gene (A, B and C) from each parent, that individual therefore has up to six distinct HLA Class I molecules expressed on the surface of their cells (Table 1).
Unlike almost every other cell type (except B cells), T cells do not have the exact same chromosomal DNA sequences as other cells in the body. As T cells develop, chromosomal DNA encoding the TCR of the CD8 T cells of each individual is rearranged and ‘educated’ to respond only to peptides presented in the context of a specific HLA Class I molecule present in that individual. This produces a great advantage in infectious disease, as it becomes difficult for a virus to adapt and spread from person to person, because each person’s HLA array is genetically distinct. Conversely this diversity presents a serious technical challenge for organ and tissue transplantation: T cells will naturally attack and reject tissue from a genetically distinct donor, expressing “non-self” HLA. As described below, it also presents challenges and opportunities for TCR-based therapeutics.
A tumour cell expresses numerous proteins, and because the HLA peptide‑presentation system is very efficient, the universe of peptides displayed on the surface of a tumour cell for TCR recognition is very large. However, most of these peptides may also be presented by normal cells. Therefore, therapeutically useful tumour antigens represent a tiny fraction of the genes expressed by a tumour cell. To be therapeutically useful, antigenic peptides must be presented in a way that allows immune responses to destroy cancer cells without causing unacceptable damage to healthy tissue. Many potential candidate antigens are under consideration. Examples of well‑studied candidates include NY‑ESO-1 and MAGEA4 (expressed in many cancer types and not in healthy adult tissue), and HPV E7 (expressed in many tumours caused by infection with human papilloma virus).
In an NY-ESO-1+ tumour cell, fragments of the NY-ESO-1 protein are processed into short peptides and loaded onto Class I HLA molecules for presentation at the cell surface. In an HLA-A*02:01+ individual, some NY-ESO-1 peptides will be presented in the context of HLA-A*02:01 for recognition by HLA-A*02:01-restricted T cells. The first NY‑ESO-1-specific T cells characterised in the lab were derived from human donors that were cancer survivors. The genetic sequence of TCRs derived from those and later experiments helped lay the groundwork for the field of TCR therapeutics.
In previous decades, isolation, sequencing and characterisation of DNA encoding a specific TCR was laborious and technically challenging. Using modern methods of cell sorting, next‑generation sequencing, artificial intelligence and synthetic biology, it is now feasible to isolate, analyse and engineer TCR sequences for commercial therapeutic purposes with much greater efficiency. Some examples of new products incorporating TCR and TCR-like technologies are below.
Soluble TCRs and TCR Mimics: In the natural context, TCRs are always expressed as transmembrane proteins incorporated into the plasma membrane of T cells. Using synthetic biology, it is possible to engineer soluble TCR molecules that are unattached to a cell, like monoclonal antibodies with respect to manufacturing and therapeutic use. With convergent technology, it is possible to engineer monoclonal antibodies that mimic the specificity of TCRs for tumour‑specific pHLA targets. These biologics can be functionalized with the same array of immunotoxins, radiological agents, and immune effectors currently deployed as monoclonal antibody therapeutics.1
TCR-based T-cell engagers: Among the many options for functionalisation of soluble tumour-binding agents, an especially promising approach is to link the tumour-binding agent to a second domain that binds to and activates T cells irrespective of a T cell’s original specificity. This approach allows in vivo redirection of T-cell responses, causing local T cells to attack a tumour expressing target pHLA.2
TCR-engineered Immune Effector Cells: Like CAR T cells, T cells may be engineered to express artificial TCRs directed to a target tumour pHLA. The potential for this category of immune effectors to treat cancer is a wide-open prospect, and can engage the same range of technologies as is being applied in the CAR field. These include complex logic‑gated control of effector cell activity, involvement of multiple effector cell types, and in vivo gene transfer to bypass ex vivo manufacturing steps.3
Conclusion
Human evolution has developed an elegant system that allows HLA presentation to open a ‘window into the cell’ and enable very precise immune monitoring of cellular health and disease states. As new higher‑throughput methods of discovery and biologic drug development come online, we can expect TCR-based therapeutics to play an important role in the treatment of cancer and other diseases in the coming years.
About the author
Daniel Kavanagh, PhD, RAC
Senior Scientific Advisor, Gene Therapies, Vaccines and Biologics at WCG
Dr Kavanagh engages with sponsors, CROs and research institutions on topics related to human gene transfer research. Previously, as a member of the faculty at Harvard Medical School, Dr Kavanagh was engaged in preclinical and clinical development of cellular immunotherapy and products.
References
- Robinson R, McMurran C, McCully M, Cole D. Engineering soluble T-cell receptors for therapy. The FEBS Journal 288:6159–6173 (2021).
- Froning K, Sereno A, Huang F, Demarest S. Generalizable design parameters for soluble T cell receptor-based T cell engagers. Journal for ImmunoTherapy of Cancer 10:e004281 (2022).
- Schafer P, Kelly L, Hoyos V. Cancer Therapy With TCR‑Engineered T Cells. Frontiers in Immunol, Mar 3:13:835762 (2022).
Related topics
Research & Development, T cells
Related conditions
Cancer