Ion channels: Small molecules versus biologics – the quest for the ideal Nav1.7 inhibitor
Posted: 21 September 2015 | Martin Perkins (National Research Council of Canada), Marzia Martina (National Research Council of Canada)
Ion channels modulate and control many fundamental physiological processes in various tissues and alterations in their functions give rise to a wide range of pathophysiologies, which makes them important drug targets. Indeed, for decades, drugs modulating ion channel activity have been targeted by the pharmaceutical industry. Historically, however, developing drugs targeting this protein class has been difficult, the most challenging aspect being the identification of an appropriate drug lead with desirable selectivity. This has been a particular issue for small molecules targeting ion channels.
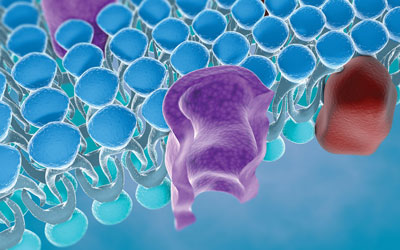
However, with the development of high-throughput automated patch-clamp technologies and the advancement in knowledge about the structure and dynamic conformation of ion channels, there has been a renewed interest in ion channel drug development. In the last decade, biologics has grown as a new class of highly effective therapeutics against difficult targets. Here, we will use the example of the strategies used to target the voltage-gated sodium channel Nav1.7 in pain and cancer indications, as a case of study to illustrate the pros and cons of developing small molecules versus antibodies as therapeutics against ion channels.
Ion channels are proteins that form pores in cell membranes and regulate the flux of ions between the intracellular and extracellular spaces of cells. They are involved in the control of many fundamental physiological processes in various tissues, and alterations in their functions give rise to a wide range of conditions, including neurological disorders, diabetes, cancer, hypertension and arrhythmia. There are ~60 different inherited ion channel diseases, known as ‘channelopathies’, that have been identified across cardiovascular, neuronal, neuromuscular, musculoskeletal, metabolic and respiratory systems, and this number is destined to grow as knowledge about ion channel functions increases. Consequently, drugs modulating ion channel activity are of great interest for the pharmaceutical industry.
The voltage-gated sodium channels (Navs) are known to be essential for the initiation and propagation of electrical signals in excitable cells. Nine pore-forming α subunits of such channels, Nav1.1-Nav1.9, have been identified in mammals1. Nav1.1–Nav1.3 and Nav1.6–Nav1.9 play major roles in neurons, whereas Nav1.4 is the muscle sodium channel and Nav1.5 is the predominant cardiac myocyte channel. Navs have also been found to have relatively high expression levels in cell types that are considered non excitable, including immune cells, fibroblasts and cancer cells. In particular, Navs are functionally expressed in many types of carcinomas (breast, cervix, colon, lung, skin, ovary and prostate) where they appear to accelerate the development of cancer metastase3.
Nav 1.7 channel: the challenge
Nav1.7 has been extensively recognised by genetic, structural and functional studies as having a central role in pain signalling in humans5. Genetic studies show that mutations in the SCN9A gene which encode for Nav1.7 produce familial pain disorders7. These mutations can be described as gain of function mutations (increase activity of Nav1.7) which cause paroxysmal extreme pain disorder (PEPD) or loss of function mutations (inhibition of Nav1.7) which are linked to complete insensitivity to pain (CIP)5.
Recently, Nav1.7 has also been found to be over-expressed in certain human cancers6, i.e., genetic studies show that SCN9A is up-regulated in human prostate cancer8. Although the cellular mechanisms that regulate Nav expression and activity in metastatic cells remain unclear4, there is evidence that expression of Nav1.7 is auto-regulated by activity-dependent positive feedback which dominates the effect of different growth factors6. However, despite the growing evidence of the involvement of Nav in cancer, development of treatment using Navs inhibitors is still at an early stage3.
Table 1: Differences between small molecules and biologics
|
Small Molecules |
Biologics |
Size |
500-1000 Da |
150-160 kDa (mAb) |
Target specificity/safety |
Low/non-specific: · Toxicity generally non-specific to target (concern due to off targets activity) · No potential for immunogenicity |
High: · Toxicity related to the target (usually expected) · Potential for immunogenicity |
Stability |
Stable |
Unstable, sensitive to external condition. |
Structure/Characterization |
Simple and well-defined structure. The active ingredient has a chemical structure. Completely characterized. |
Complex, heterogeneous structure, defined by the manufacturing process. Impossible to fully characterize the molecular composition. |
Manufacturing |
Chemical synthesis (less expensive). A lot of identical copies can be produced. |
Produced by living cell cultures (more expensive). Control is difficult and is difficult to ensure identical copies. |
Administration/distribution |
Usually oral Extensive distribution in the body (cross the brain-blood barrier) |
Mostly intravenous Limited distribution within the body (do not cross the brain blood barrier) |
The first generations of molecules developed against Navs were analgesics. These drugs were clinically effective, but, due to their non-selective Nav activity, their analgesic potential was limited by numerous side effects (dizziness, sedation, convulsions and cardio-toxicity). More recently it has been shown that in vitro application of the potent Navs blocker tetrodotoxin (TTX) in prostate cancer cells inhibits cancer invasion, proliferation and migration9,10. Likewise, in vivo injection of TTX directly into primary tumours, to avoid systemic toxicity of TTX (Copenhagen rat model of prostate cancer), reduced the number of lung metastasis by >40%11.
In the past few decades pharmaceutical companies have implemented a large number of programs to develop a second generation of Nav inhibitors which target specific subunits with a high degree of specificity in order to develop more effective and better tolerated compounds for pain management. However, the development of a novel therapeutic selectively targeting Nav1.7 represents a challenge due to the high degree of amino acid sequence homology among the different Nav subtypes1. In addition, Navs are complex structures which have different dynamic states (closed, open or inactivated). Nevertheless, large bodies of evidence concerning the sequences containing the lower homology between the subtypes of human Nav channels, regions containing mutations causing CIP5 and interaction regions for pore blockers (i.e., small molecules and natural toxins) are now available to help with the development of new inhibitors. Structural information associated with multiple function-related conformations of Nav channels is also indispensable for understanding the underlying molecular mechanism of gating12. This knowledge has been used to design strategies for the development of both small molecules – classic drugs developed by pharmaceutical companies – and biologics (a class of drugs based on proteins) as therapeutics for pain that targets Nav1.7.
The selectivity conundrum
The last 10 years have seen the advent of new automated synthesis methods in research laboratories that enable chemists to develop a lot of new and more selective small molecules (for review see 13). In addition, the advent of the high-throughput automated patch-clamp systems (HT-APS) in ion channel drug discovery has revolutionised the world of small molecule screening. HT-APS enables compound profiling, lead optimisation, secondary screening, primary screening of focused/targeted libraries and assay development of numerous compounds on many types of ion channels and cellular models. This has resulted over the past four years in a continuous effort in the discovery of new Nav1.7 blockers by a large number of pharmaceutical companies14. Nevertheless, it is still a challenge to develop subtype-selective compounds with small molecules. Conversely, biologics (monoclonal antibodies (mAbs) in particular) can be produced to target-specific subunits or epitopes or state of a channel with a high degree of specificity (for the difference between small molecules and biologics see Table 1; page 00). mAbs have the great advantage of being extremely selective, however, the majority of mAbs developed against ion channels so far are binders lacking functional efficacy.
The difficulty in producing functional mAbs is down to these targets having multiple transmembrane regions and small extracellular loops, as well as being tricky to purify (due to unstable antigens) and having functional epitopes that are generally inside the pores. However, the tarantula toxin ProTx-II, a 30 amino acid peptide which is a highly selective Nav1.7 inhibitor15, does not bind the Nav1.7 pore region, demonstrating that targeting molecular regions outside the pore could be a successful strategy in identifying subtype-selective compounds. In addition, another type of biologic has been investigated – the single-domain antibody (sdAb) – as an approach to bind to epitopes not accessible to whole antibodies. sdAbs represent the smallest antibody with diagnostic and therapeutic use. They are antibody fragments engineered from the heavy-chain antibodies generated in camelids and consist of a single monomeric variable antibody domain with a molecular weight of only 12–15 kDa but retain full antigen binding capacity with high target specificity and affinity.
Biologics have the potential for much greater selectivity than small molecules. Recently, the novel, potent but nonselective Nav1.7 channel blocker XEN-402 significantly reduced the amount of pain experienced by patients suffering from inherited erythromelalgia16. Two additional small molecules (ICA-121431 and PF-04856264) targeting a novel binding site within the Nav voltage sensor have been shown to be potent Nav1.1, Nav1.3, Nav1.6 and Nav1.7 inhibitors with a remarkable selectivity over cardiac Nav1.5 channels (~1000-fold)17. There are small molecule Nav1.7 inhibitors in phase II clinical trials which are well tolerated with no serious adverse events (see 13) suggesting that an acceptable degree of selectivity can be achieved with small molecules.
With respect to biologics, Lee and colleagues have generated a highly selective and potent inhibitory mAb against Nav1.718. They looked at the crystal structure of the bacterial NaV channel NaVAb12 and identified a sequence of amino-acids in the region of the voltage-sensor paddle that was critical for the channel functionality18. In patch-clamp studies, the antibody raised against this sequence of amino-acids (peptide) showed high selectivity for Nav1.7 and low selectivity for Nav1.1-1.6 and Nav1.8. This antibody was antinociceptive in mice models of inflammatory and neuropathic pain after intravenous and intrathecal administration.
Selectivity is particularly relevant with regard to safety and small molecules can exhibit off-target toxicities which limits the therapeutic dose. This is rarely an issue with biologics due to their very high selectivity. However, biologics do have the potential for immunogenicity which can limit their dose and/or dosing regimen, although strategies such as humanisation of the mAb exist, and minimise this risk.
The ideal therapeutic
A successful therapeutic must also satisfy other factors such as administration methods/regime, patient compliance and production costs. Small molecules are generally administrated orally with a large volume of distribution whilst biologics are typically administered intravenously with a limited distribution (Table 1; page 00). Data from systemic and intrathecal administration of the Nav1.7-specific mAb developed by Lee and collaborators seem to suggest that Nav1.7-mediated antinociception is obtained via both peripheral and central mechanisms18. However, since the majority of therapeutics, biologics and small molecules do not penetrate the blood-brain barrier (BBB) sufficiently to induce pharmacologically meaningful effects on central nervous system (CNS) targets18, a successful therapeutic could be developed by increasing brain penetration. This could be achieved by engineering antibody-drug-conjugates (ADC) or bi-specific antibodies in which one antibody binding specificity recognises a BBB receptor that undergoes receptor-mediated transcytosis (RMT) from the brain into the CNS, and the second binding specificity recognises a therapeutic target, i.e., Nav1.7 within the CNS.
The development of BBB-crossing bi-specific antibodies requires targeted antibody engineering to optimise multiple characteristics of ‘BBB carrier’ and therapeutic arms, as well as other antibody properties impacting pharmacokinetics and effector function19. It is widely recognised now that the preclinical models of chronic pain do not translate well to the clinic with many failures with compounds that had good efficacy in the animal models. The possibility of targeting an additional CNS site may provide the additional efficacy required to be a successful analgesic. With respect to a potential cancer indication, the development of a bi-specific Ab targeting both the tumour cells and Nav1.7 could ensure concentration of the therapeutic Ab in the tumour.
mAbs are the fastest growing category of therapeutics. Between 2005 and 2014, 55 biologic new molecular entities (NMEs) were approved, accounting for 20% of total NME approvals in this time period. In 2014, the US Food and Drug Administration’s Center for Drug Evaluation and Research issued 11 biologic NME approvals, the highest total for a single year21. However, the cost of biologics is high due to the complexity of their structure and production methods. Biologics can cost up to 20 times more than regular drugs, with some costing $100,000 a year. In the absence of an effective and safe small molecule therapeutic this is clinically and commercially acceptable but it becomes a factor if a small molecule equivalent exists.
Concluding remarks
In conclusion, the key factor in developing a potential therapeutic targeting ion channels and Nav1.7 in particular is selectivity. Historically, this has proven to be a major challenge for small molecules and is an area that biologics, particularly Abs, may well have an advantage. With respect to pain, the latest generation of small molecule inhibitors is in the clinic. If these are efficacious without the side-effects seen with the first generation inhibitors then it is perhaps difficult to see where there would be an advantage of a biologic. If CNS access is required for efficacy in man then a BBB-crossing mAb targeting Nav1.7 may have such an advantage. With respect to a cancer indication, a bi-specific Ab targeting both the tumour cell and Nav1.7 may have an advantage in allowing lower systemic exposure due to concentration within the tumour and thus avoiding side-effects associated with inhibiting the normal functioning of the Nav1.7 ion channel.
References
- Ashcroft, FM. From molecule to malady. Nature 440, 440-447 (2006)
- Catterall, WA, Goldin, AL, Waxman, SG International Union of Pharmacology. XLVII. Nomenclature and structure–function relationships of voltage-gated sodium channels. Pharmacological Reviews, 2005; 57, 397–409
- Litan, A, Langhans, SA. Cancer as a channelopathy: ion channels and pumps in tumor development and progression. Frontiers in Cellular Neurosciences. 2015; 9, 88 doi: 10.3389/fncel.2015.00086
- Ding, Y, Brackenbury, WJ, Onganer, PU, Montano, X, Porter, LM, Bates, LF, Djamgoz, MB. Epidermal growth factor upregulates motility of Mat-LyLu rat prostate cancer cells partially via voltage-gated Na+ channel activity Journal of Cellular Physiology. 2008; 215, 77-81
- Dib-Hajj SD, Cummins TR, Black JA, Waxman SG. Sodium channels in normal and pathological pain. Annual Review in Neuroscience. 2010; 33, 325–347
- Fraser, SP, Ozerlat-Gunduz, I, Brackenbury, WJ, Fitzgerald, EM, Campbell, TM, Coombes, RC, Djamgoz MBA. Regulation of voltage-gated sodium channel expression in cancer: hormones, growth factors and auto-regulation. Philosophical Transactions of the Royal Society B: Biological Sciences. 2014; 369(1638):20130105 doi:10.1098/rstb. 2013.0105
- Cox, JJ, Reimann, F, Nicholas, AK, Thornton, G, Roberts, E, Springell, K, Karbani, G, Jafri, H, Mannan, J, Raashid, Y, Al-Gazali, L, Hamamy, H, Valente, EM, Gorman, S, Williams, R, McHale, DP, Wood, JN, Gribble, FM, Woods, CG. An SCN9A channelopathy causes congenital inability to experience pain. Nature. 2006; 444, 894-898
- Diss, JK, Fraser, SP, Walker, MM, Patel, A, Latchman, DS, Djamgoz, MB. Beta‑subunits of voltage‑gated sodium channels in human prostate cancer: quantitative in vitro and in vivo analyses of mRNA expression. Prostate Cancer and Prostatic Diseases, 2008, 11, 325‑333
- Laniado, ME, Lalani, EN, Fraser, SP, Grimes, JA, Bhangal, G, Djamgoz, MB, Abel, PD. Expression and functional analysis of voltage‑activated Na+ channels in human prostate cancer cell lines and their contribution to invasion in vitro. American Journal of Pathology, 1997; 150, 1213‑1221
- Grimes, JA, Fraser, SP, Stephens, GJ, Downing, JE, Laniado, ME, Foster, CS, Abel, PD, Djamgoz, MB. Differential expression of voltage‑activated Na+ currents in two prostatic tumour cell lines: contribution to invasiveness in vitro. FEBS Letters. 1995; 369, 290‑294
- Yildirim S, Altun S, Gumushan H, Patel A, Djamgoz MBA. Voltage-gated sodium channel activity promotes prostate cancer metastasis in vivo. Cancer Letters, 2012; 323, 58–61
- Payandeh, J, Scheuer, T, Zheng, N, Catterall, WA. The crystal structure of a voltage-gated sodium channel. Nature. 2011; 475, 353–358
- De Lera Ruiz M, Kraus RL. Voltage-Gated Sodium Channels: Structure, Function, Pharmacology,and Clinical Indications. Journal of Medical Chemistry, ahead of publication DOI: 10.1021/jm501981g
- Zuliani, V, Rapalli, A, Patel, MK, Rivara, M. Sodium channel blockers: a patent review (2010- 2014). Expert Opinion Therapeutic Patents, 2015, 25, 279-290
- RE, Warren, VA, Kraus, RL, Hwang, JC, Liu, CJ, Dai, G, Brochu, RM, Kohler, MG, Gao, YD, Garsky, VM, Bogusky MJ, Mehl, JT, Cohen, CJ, Smith, MM. Two tarantula peptides inhibit activation of multiple sodium channels. Biochemistry. 2002; 41, 14734-14747
- Goldberg, YP, Price, N, Namdari, R, Cohen, CJ, Lamers, MH, Winters, C, Price, J, Young, C E, Verschoof, H, Sherrington, R, Pimstone, SN, Hayden, MR. Treatment of Nav1.7-mediated pain in inherited erythromelalgia using a novel sodium channel blocker. Pain; 2012, 153, 80-85
- McCormack, K, Santos, S, Chapman, ML, Krafte, DS, Marron, BE, West, CW, Krambis, M J, Antonio, BM, Zellmer, SG, Printzenhoff, D, Padilla, KM, Lin, Z, Wagoner, PK, Swain, NA, Stupple, PA, De Groot, M, Butt, RP, Castle, NA. Voltage sensor interaction site for selective small molecule inhibitors of voltage-gated sodium channels. Proceeding National Academy of Science USA, 2013; 110 (29), E2724-2732
- Lee JH, Park CK, Chen G, Han Q, Xie RG, Liu T, Ji RR, Lee SY. A monoclonal antibody that targets a NaV1.7 channel voltage sensor for pain and itch relief. Cell; 2014, 157, 1393-1404. doi: 10.1016/j.cell.2014.03.064
- Arrowsmith, J, Miller, P. Trial watch: phase II and phase III attrition rates 2011-2012. Nature Review Drug Discovery. 2013; 12, 569
- Stanimirovic, D, Kemmerich, K, Haqqani, AS, Farrington, GK. Engineering and pharmacology of blood-brain barrier-permeable bispecific antibodies. Advances in Pharmacology. 2014; 71, 301-35; doi: 10.1016/bs.apha.2014.06.005
- Miller KL, Lanthier M. Regulatory watch: Innovation in biologic new molecular entities: 1986-2014. Nature Reviews Drug Discovery. 2015; 14, 83; doi:10.1038/nrd4535
Biographies
Dr Marzia Martina is the leader of the Neurophysiology Team within the Department of Translational Biosciences at the National Research Council of Canada. Her work focuses on the development of therapeutic antibodies targeting ion channels, as well as physiologically-relevant CNS models to evaluate therapeutics. She holds a PhD in Human Physiology from the University of Milan where she investigated the modulatory effects of dopamine on HVA calcium channels in sensory neurons. She studied the physiological and networking properties of central and intercalated neurons in the amygdala at Laval University, Quebec City, during her postdoctoral fellowship and, before joining the NRC, she was Assistant Professor at the Department of Psychiatry (University of Ottawa) conducting electrophysiological studies on the effects of D-amino acids on NMDA receptor function. Contact her at: [email protected]
Dr Martin Perkins obtained his PhD in neuropharmacology in 1979 from the University of London. Currently he is a Principal Research Officer in the National Research Council of Canada. His research is aimed at developing potential biological therapeutics for both peripheral and central applications. He has had over 25 years’ experience in multinational pharmaceutical companies with a focus on developing novel analgesics for chronic pain. He has experience of the entire preclinical development pathway including taking candidate drugs to First Time in Man.
Related topics
Drug Targets, Ion Channels
Related conditions
Diabetes
Related organisations
National Research Council of Canada
Related people
Martin Perkins, Marzia Martina