From fibroblasts to medicinal signalling cells: a paradigm shift in translational stem cell research
Posted: 20 July 2018 | Darius Widera, Jonathan Sheard, Thamil Selvee Ramasamy | No comments yet
Mesenchymal stem cells (MSCs) are fibroblast-like cells that give rise to mesenchymal derivatives including bone, fat and cartilage cells. In addition to the bone marrow, they can be readily obtained from adult vascularised peripheral tissues including adipose, olfactory, respiratory tissue, and peripheral blood.
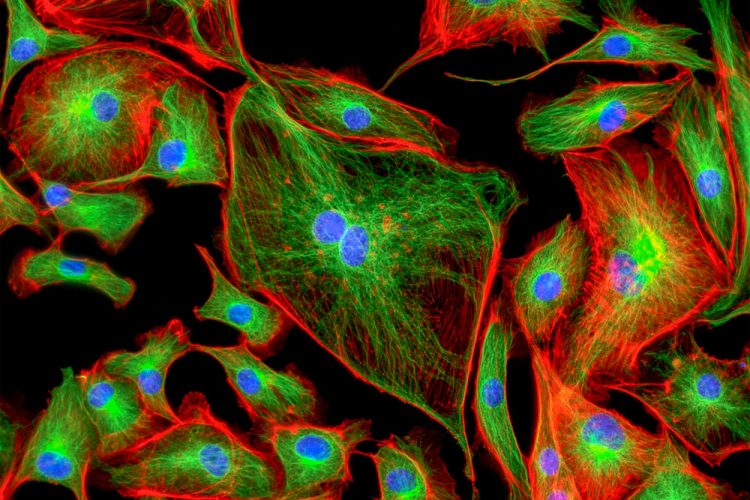
MSCs and MSC-like cells can be isolated from the amniotic fluid and neonatal tissues such as umbilical cord and placenta. In general, MSCs are well tolerated, non-tumourigenic and the transplantation of these cells appears to be beneficial in a variety of diseases and conditions. Their high availability and relatively low-risk profile made MSCs a promising stem cell source for clinical exploitation.1 As of May 2018, more than 843 clinical trials have been registered on the ClinicalTrials.gov database with indications including diabetes, cancer, cardiovascular disorders, and musculoskeletal symptoms. Initially, it has been postulated that transplanted MSCs exhibit their therapeutic potential through interaction and differentiation into cells and tissues affected by the respective condition. Going even further, several in vitro studies claimed that MSCs can cross the germ layer boundary and give rise to neuronal (ectodermal) and pancreatic islet-like cells (endoderm).
However, most pre-clinical transplantation studies demonstrated negligible numbers of surviving MSCs in the host tissue and lack of differentiation into non-mesodermal cell types. Nowadays, it is widely accepted that MSCs lack the ability to generate progeny beyond bone, fat, and cartilage cells and that their level of engraftment after transplantation is extremely low. Nevertheless, MSCs have been unequivocally shown to mediate beneficial effects in many animal models of disease and in clinical conditions. The current understanding of their mode of action includes: a reduction of inflammation, immune-modulation, and an increase of the endogenous regeneration of the host tissue. Moreover, all these `bystander effects` are mediated by paracrine factors secreted by the MSCs. Briefly, the paracrine factors can be soluble or embedded within nano-scale extracellular vesicles. In the following, we discuss the journey from bone marrow-derived fibroblast-like cells to the modern understanding of MSCs as medicinal signalling cells and, critically, discuss the clinical and commercial impact of the factors secreted by these cells.
Fibroblast colony-forming units
The suggestion of a stem cell-like population as the origin of non-haematopoietic bone marrow cells, which contribute to wound repair and have a fibroblastic-like morphology, was made almost 150 years ago by the German-Jewish Pathologist, Julius Friedrich Cohnheim.2,3The subsequent work conducted by the Russian Scientist, Alexander Friedenstein, from the late 1960s through the 1970s, credited him with the discovery of MSCs. He made the critical observation that the bone marrow, in postnatal life, is a reservoir of stem cells for mesenchymal tissues. Briefly, he isolated adherent, clonogenic, fibroblast-like cells from the rodent bone marrow, termed them ‘fibroblast colony-forming units’ and demonstrated that these cells possess high replicative potential in vitro (CFU-F).4-7 Later, CFU-F were shown to be able to undergo mineralisation in vitro8,9 and to have osteogenic differentiation potential in vivo.10
Mesenchymal stem cells
In 1991, based on the embryonic perspective of multipotent progenitor cells located within the mesodermal layer, Caplan postulated that a population of mesenchymal cells able to give rise to a spectrum of mesenchymal cells is present in adult bone marrow.11 Indeed, in 1999, Pittenger verified this hypothesis by showing that cells isolated from human adult bone marrow aspirates display a stable phenotype in an in vitro monolayer culture and can be induced to differentiate into the osteogenic, adipogenic, and chondrogenic lineages (Figure 1).12 This multi-lineage differentiation potential led to the assumption that this stromal cell population has stem cell properties. Consequently, MSCs have been termed mesenchymal stem cells.
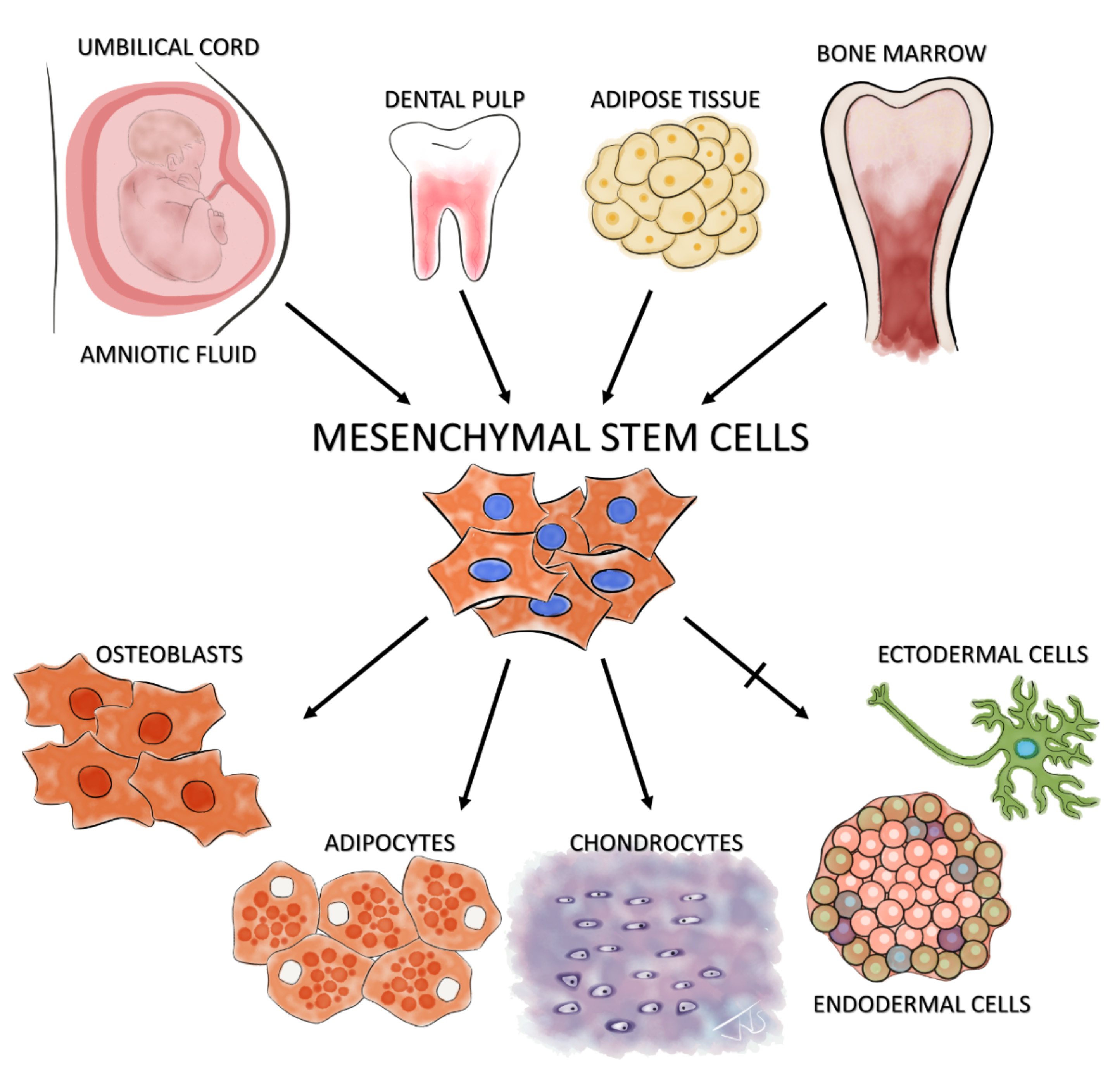
Figure 1: Isolation and differentiation potential of MSCs The illustration shows examples of known niches of MSCs – the amniotic fluid, umbilical cord, dental pulp, adipose tissue, and bone marrow. The standard ISCT minimal criteria of tri-lineage differentiation of these isolated MSCs along osteoblastic, adipogenic and chondrogenic lineages is also shown. The controversial claim of MSCs’ potential to cross the germ layer boundaries and differentiate into ectodermal (neuron-like cells) and endodermal cells (pancreatic-islet like cells) is shown with a crossed arrow
Mesenchymal stromal cells
In 2006, to standardise the nomenclature and to allow for a comparison of scientific studies among different laboratories, the International Society for Cellular Therapy (ISCT) introduced a set of minimal criteria for MSCs. According to this definition, MSCs must be plastic adherent, able to differentiate into adipogenic, chondrogenic and osteogenic cells, and should express CD105, CD73 and CD90 cell-surface markers. Moreover, MSCs are defined by the lack of the hematopoietic markers CD45, CD34, CD14, CD11b, CD79α, CD19, and HLA-DR.13 More importantly, in this position paper, the ISCT recommended the use of term multipotent mesenchymal stromal cells (MSC) instead of mesenchymal stem cells. Notably, this change in nomenclature has not been justified by arguments addressing the stem cell characteristics of MSCs.
MSCs: more than multipotent?
In the following years, a multitude of studies suggested that MSCs might be able to cross the germ layer boundaries and differentiate into ectodermal and endodermal cells. In particular, neuronal differentiation has been claimed by several independent laboratories.14-18 However, most of the studies relied heavily on morphology and marker expression and did not provide functional data in direct comparison to neurons. Notably, MSCs display a neuron-like morphology after exposure to cellular stress and express neuronal markers even after differentiation into osteogenic and adipogenic fate.19 The lack of neuronal differentiation has also been supported by several studies showing no functional integration and differentiation of MSCs in the brain tissue. Similarly, it has been postulated that MSCs could undergo endodermal differentiation into pancreatic islet-like cells.15,20 However, the authors did not provide functional proof of differentiation. Despite several attempts to prove cross-lineage differentiation, there is little evidence for the claim that MSCs can give rise to more than mesenchymal cells without direct programming using forced expression of ectodermal and endodermal factors. This relatively low differentiation potential and the negligible level of engraftment contrast with the promising pre-clinical and clinical results after MSC transplantation. Briefly, MSCs have been shown to alleviate symptoms of a broad spectrum of diseases and symptoms including liver cirrhosis (affected germ layer: endoderm),21 severe ischaemic heart failure (affected germ layer: mesoderm),22 and progressive Multiple Sclerosis (affected germ layer: ectoderm).23 This discrepancy led to the conclusion that the therapeutic effects of MSC-administration are a result of paracrine bystander effects rather than a consequence of an engraftment and differentiation towards lost cell types.24-29
Medicinal signalling cells
The prominent bystander effects mediated by MSCs led to a newer definition describing these cells as ‘Medicinal signalling cells.30 According to this definition, MSCs function as surveyors of their microenvironments. During local injury, they are released from their niche, become activated, and establish a regenerative microenvironment by secreting bioactive molecules and regulating the local immune response. If transplanted into a side of injury or degeneration, MSCs sense the injury signals and release paracrine factors contributing thereby to the endogenous regeneration process in the host. These trophic and immunomodulatory activities suggest that MSCs may indeed serve as site-regulated `drugstores` in vivo.31,32 This behaviour can be explored industrially, where cultivated MSCs can be used as `biofactories` to produce paracrine factors that can be used as a potential substitute for cell transplantation.
Secretome of MSCs as an emerging alternative to MSC transplantation
The regenerative competency of MSCs is most likely mediated by paracrine factors within the MSC-secretome, as described above. This is evidenced by a similar tissue repair and regeneration potential of MSC-derived conditioned medium (CM) compared with MSC transplantation.33-35 In the following, we briefly discuss the active compounds within the CM focusing on soluble proteins and the cargo of extracellular vesicles (EVs) released by MSCs.
Soluble factors
Secretion of soluble factors is at least partly responsible for the two key therapeutic modes underlying tissue repair mechanisms mediated by MSCs, namely: immunosuppressive / modulatory and tissue regeneration effects. Notably, MSCs do not confer an immunosuppressive effect in a constitutive manner, but rather in response to the local tissue microenvironment. More specifically, MSCs are able to sense inflammation and tissue damage via tumour necrosis factor receptors (TNFRs)36 and toll-like receptors (TLRs),37,38 resulting in the release of an array of soluble factors including human leukocyte antigen G (HLA-G), IL-6, IL-10, indoleamine 2,3-dioxygenase (IDO), nitric oxide (NO) and TGF-β. These soluble factors elicit immunosuppressive and immunomodulatory actions by regulating the proliferation and function of a variety of immune cells.39,40
A broad spectrum of cytokines, chemokines and growth factors (EGF, TGF-β, IGF-1, HGF, VEGF) secreted by MSCs are also known to stimulate molecular and cellular processes involved in regeneration, including proliferation of host cells, angiogenesis, chemo-attraction and tissue remodelling. Interestingly, in vitro exposure of MSCs to pro-inflammatory factors (licencing) has been shown to increase the regenerative potential of MSCs.41 Moreover, it has been demonstrated that relatively high levels of anti-apoptotic factors are present in bone marrow MSC-CM and that these soluble factors protect pancreatic cells from apoptosis in a diabetic animal model.42 Intriguingly, CM of adult periodontal ligament MSCs from young donors was shown to increase proliferation of aged MSCs in vitro and in vivo. This effect has been largely attributed to secreted soluble growth and differentiation factors including IGF-1, PDGF, IL-1, TGF-β, BMP-2 and BMP-4.
Neither single soluble factors alone nor combinations thereof can fully recapitulate the therapeutic effects of MSCs. This can be explained by the fact that MSCs act as `cytokine biofactories` that adapt their products in response to external cues. Moreover, over the past decade, the paracrine effects of MSCs have also been shown to be at least partly mediated by EVs.
Extracellular vesicles
The term `extracellular vesicle` is an umbrella term for a heterogeneous population of vesicles secreted by all animal and human cell types. At least two types of these vesicles, exosomes44 and microvesicles,45 have been shown to contribute to the regenerative potential of MSC-secretome. Briefly, exosomes are nanoscale vesicles of endosomal origin with a size ranging from 30 to 100nm, while microvesicles bud from the plasma membrane and are slightly larger (100–100nm) (Figure 2).46 With the existing technologies, microvesicles and exosomes can hardly be distinguished. Consequently, the International Societyof Extracellular Vesicles (ISEV) suggested using the term EV for both types of vesicles.46 Currently, differential centrifugation represents the most widely used method for EV isolation.47,48 This method includes centrifugation at low speed to remove cell debris and high-speed ultracentrifugation to purify EVs. However, it has been reported that ultracentrifugation can negatively affect the integrity of exosomes.49 Despite the lack of standard isolation methods, EVs have been shown to harbour a regenerative potential that is similar to MSC-mediated effects. Direct side-by-side comparisons in models of acute kidney failure, ischemic stroke, and lung injury revealed that MSCs and MSC-derived EVs exert comparable regenerative effects.50-53 Despite these exciting results, recent studies suggest that soluble factors and EVs affect different aspects of the regeneration process and that these two fractions might act in a synergistic manner.54 Consequently, full MSC-CM might have superior regenerative potential compared with isolated EVs or the soluble factors.
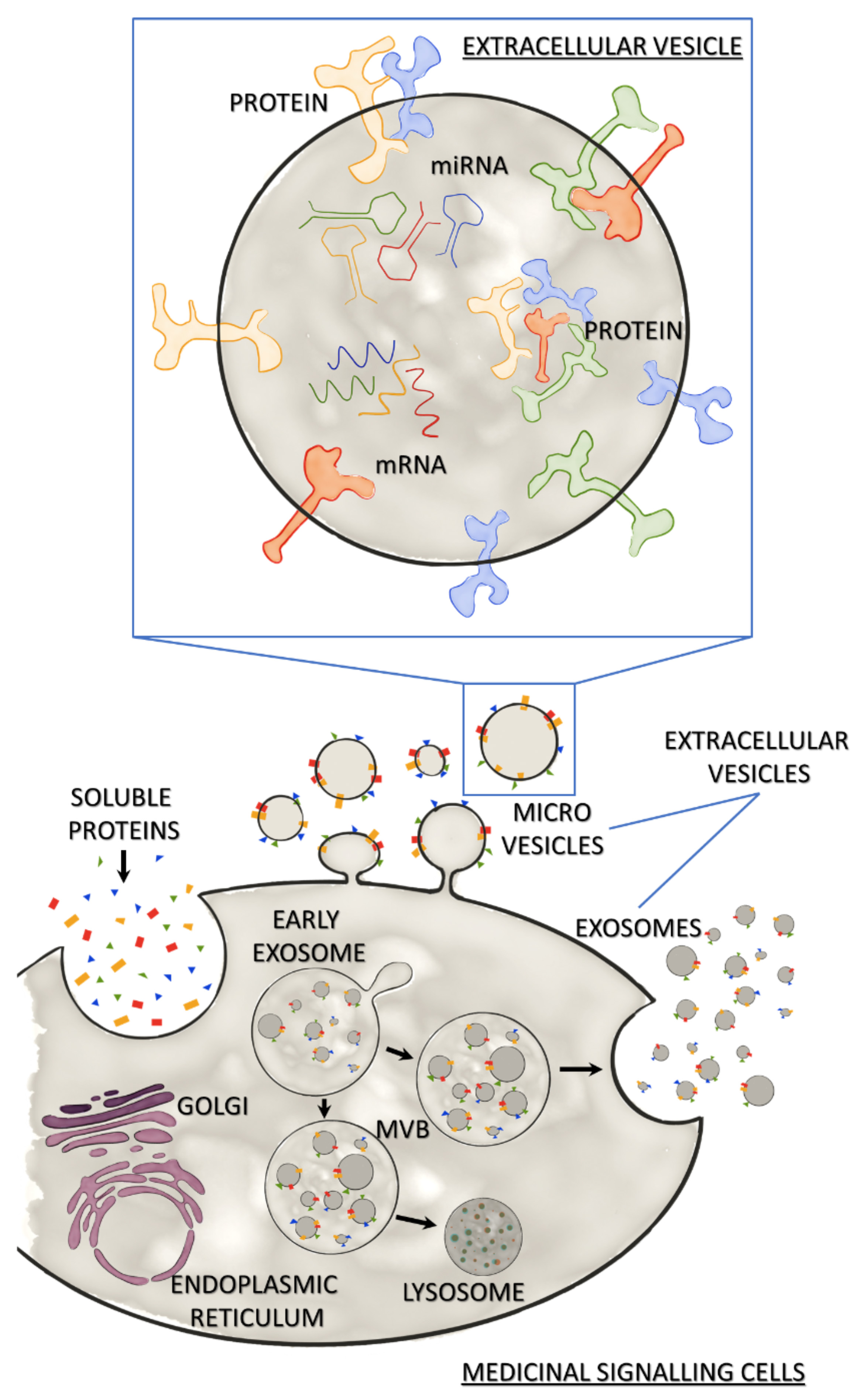
Figure 2: Medicinal signalling cells and their secretome Secretome of Medicinal Signalling Cells released in response to the local microenvironment consists of soluble secreted proteins and extracellular vesicles (EVs). MSCs release EVs via direct budding (microvesicles) or the release of exosomes from multi-vesicular bodies (MVB) by exocytosis. MVBs may bind to lysosomes leading to degradation of the exosomal cargo. The expanded view of an EV shows the characteristic EV cargo (mRNA, miRNA and proteins), as well as membrane-bound proteins.
Summary and conclusions
Since their discovery in 1867, views on MSCs have undergone dramatic changes. Over the years, MSCs have been considered fibroblast-like cells, mesenchymal stem cells, multipotent marrow stromal cells, and most recently, medicinal signalling cells. Their paracrine power resulted in a paradigm shift in the field of regenerative medicine. In the current view, tissue regeneration mediated by MSCs does not depend on their differentiation capacity but rather on the release of soluble factors and extracellular vesicles, which together facilitate the endogenous regeneration process. This represents an exciting clinical and commercial avenue. The use of the secretome to substitute stem cell transplantation has a significantly lower risk profile (no rejection, no tumour formation) and is more cost-efficient, but also poses several challenges. The MSC-secretome is a biological with a poorly defined composition making regulatory affairs very challenging. In addition, there is high need of standardisation of the production process and the development of appropriate bioactivity assays to test the anti-inflammatory, immunomodulatory and proangiogenic potential.
Moving forward in developing this strategy, optimal culture conditions, sources of MSCs and pre-conditioning of cells prior to harvesting the CM need to be explored for an effective therapeutic design that can be commercially exploited. Meanwhile, addressing the question of whether the EV fraction or the full secretome exhibit superior therapeutic outcomes is a hot topic in basic research that will provide new insights into cell-free regenerative medicine.
Biography
DR JONATHAN SHEARD is a postdoctoral researcher for Sheard BioTech Ltd, working to provide expertise in Biomedical Research, Stem Cell Biology and Advanced Imaging Techniques. He is currently based at the University of Reading working alongside the Widera Lab, investigating the use and development of a novel scaffold for biomedical research applications. He completed his PhD in Biomedical Sciences at Aston University, focusing on the identification and safe use of mesenchymal stromal cells towards clinical application, using high-content screening and live cell imaging systems.
DR THAMIL SELVEE RAMASAMY is a Senior Lecturer at Department of Molecular Medicine, University of Malaya, Malaysia. She also serves as the President of Tissue Engineering and Regenerative Medicine Society of Malaysia (TESMA). Her laboratory focuses on understanding and exploring therapeutic potential of pluripotent and multipotent stem cells by developing cutting-edge research strategies including tissue engineering, stem cell preconditioning, and rejuvenation strategies for development of both cell-based and cell-free approaches for their applications in regenerative medicine.
DR DARIUS WIDERA is a Lecturer in Stem Cell Biology and Regenerative Medicine at the University of Reading. His lab is interested in stem cell research and the influence of injury and inflammation on somatic cells. His team wants to understand how injury signals influence differentiation, proliferation, and migration of stem cells. They also study various adult human stem cell populations and their secretome, develop 3D cell culture methods, and work on novel stem cell-based but cell-free therapies for degenerative disorders and conditions.
References
- Lalu MM, McIntyre L, Pugliese C, Fergusson D, Winston BW, Marshall JC, Granton J, Stewart DJ, Canadian Critical Care Trials Group. (2012) Safety of cell therapy with mesenchymal stromal cells (SafeCell): a systematic review and meta-analysis of clinical trials, PLoS One. 7, e47559.
- Cohnheim J. (1867) Ueber Entzündung und Eiterung, Archiv f pathol Anat. 40, 1-79.
- Prockop DJ. (1997) Marrow Stromal Cells as Stem Cells for Nonhematopoietic Tissues, Science. 276, 71-74.
- Friedenstein A, Petrakova KV, Kurolesova A, Frolova GP. (1968) Heterotopic of bone marrow. Analysis of precursor cells for osteogenic and hematopoietic tissues, Transplantation. 6, 230-47.
- Friedenstein AJ, Chailakhjan RK, Lalykina KS. (1970) The development of fibroblast colonies in monolayer cultures of guinea-pig bone marrow and spleen cells, Cell and tissue kinetics. 3, 393-403.
- Chailakhyan RK, Fridenshtein AY, Vasil’ev AV. (1970) Clone formation in monolayer cultures of bone marrow and spleen, Bull Exp Biol Med. 69, 188-191.
- Friedenstein AJ, Deriglasova UF, Kulagina NN, Panasuk AF, Rudakowa SF, Luria EA, Ruadkow IA. (1974) Precursors for fibroblasts in different populations of hematopoietic cells as detected by the in vitro colony assay method, Exp Hematol. 2, 83-92.
- Howlett CR, Cave J, Williamson M, Farmer J, Ali SY, Bab I, Owen ME. (1986) Mineralization in in vitro cultures of rabbit marrow stromal cells, Clinical orthopaedics and related research, 251-63.
- Friedenstein AJ, Chailakhyan RK, Gerasimov UV. (1987) Bone marrow osteogenic stem cells: in vitro cultivation and transplantation in diffusion chambers, Cell and tissue kinetics. 20, 263-72.
- Ashton BA, Eaglesom CC, Bab I, Owen ME. (1984) Distribution of fibroblastic colony-forming cells in rabbit bone marrow and assay of their osteogenic potential by an in vivo diffusion chamber method, Calcified tissue international. 36, 83-6.
- Caplan AI. (1991) Mesenchymal stem cells, Journal of Orthopaedic Research. 9, 641-650.
- Pittenger MF. (1999) Multilineage Potential of Adult Human Mesenchymal Stem Cells, Science. 284, 143-147.
- Dominici M, Le Blanc K, Mueller I, Slaper-Cortenbach I, Marini F, Krause D, Dean R, Keating A, Prockop D, Horwitz E. (2006) Minimal criteria for defining multipotent mesenchymal stromal cells. The International Society for Cellular Therapy position statement, Cytotherapy. 8, 315-7.
- Jiang Y, Jahagirdar BN, Reinhardt RL, Schwartz RE, Keene CD, Ortiz-Gonzalez XR, Reyes M, Lenvik T, Lund T, Blackstad M, Du J, Aldrich S, Lisberg A, Low WC, Largaespada DA, Verfaillie CM. (2002) Pluripotency of mesenchymal stem cells derived from adult marrow, Nature. 418, 41-49.
- D’Ippolito G, Diabira S, Howard GA, Menei P, Roos BA, Schiller PC. (2004) Marrow-isolated adult multilineage inducible (MIAMI) cells, a unique population of postnatal young and old human cells with extensive expansion and differentiation potential, J Cell Sci. 117, 2971-81.
- Takeda Y, Xu Q. (2015) Neuronal Differentiation of Human Mesenchymal Stem Cells Using Exosomes Derived from Differentiating Neuronal Cells, PLoS One. 10, e0135111.
- Tropel P, Platet N, Platel JC, Noel D, Albrieux M, Benabid AL, Berge F. (2006) Functional neuronal differentiation of bone marrow-derived mesenchymal stem cells, Stem Cells. 24, 2868-76.
- Scuteri A, Miloso M, Foudah D, Orcian M, Cavaletti G, Tredici G. (2011) Mesenchymal stem cells neuronal differentiation ability: a real perspective for nervous system repair? Curr Stem Cell Res Ther. 6, 82-92.
- Foudah D, Redondo J, Caldara C, Carini F, Tredici G, Miloso M. (2013) Human mesenchymal stem cells express neuronal markers after osteogenic and adipogenic differentiation, Cell Mol Biol Lett. 18, 163-86.
- Zanini C, Bruno S, Mandili G, Baci D, Cerutti F, Cenacchi G, Izzi L, Camuss G, Forni M. (2011) Differentiation of Mesenchymal Stem Cells Derived from Pancreatic Islets and Bone Marrow into Islet-Like Cell Phenotype, PLoS ONE. 6, e28175.
- Kharaziha P, Hellstrom PM, Noorinayer B, Farzaneh F, Aghajani K, Jafari F, Telkabadi M, Atashi A, Honardoost M, Zali MR, Soleimani M. (2009) Improvement of liver function in liver cirrhosis patients after autologous mesenchymal stem cell injection: a phase I-II clinical trial, Eur J Gastroenterol Hepatol. 21, 1199-205.
- Mathiasen AB, Qayyum AA, Jorgensen E, Helqvis S, Fischer-Nielsen A, Kofoed K, Haack-Sorensen M, Ekblond A, Kastrup J. (2015) Bone marrow-derived mesenchymal stromal cell treatment in patients with severe ischaemic heart failure: a randomized placebo-controlled trial (MSC-HF trial), Eur Heart J. 36, 1744-53.
- Connick P, Kolappan M, Patani R, Scott MA, Crawley C, He X, Richardson K, Barber K, Webber DJ, Wheeler-Kingshot CA, Tozer DJ, Samson RS, Thomas DL, Du MQ, Luan SL, Michell AW, Altmann DR, Thompson AJ, Miller DH, Compston A, Chandran S. (2011) The mesenchymal stem cells in multiple sclerosis (MSCIMS) trial protocol and baseline cohort characteristics: an open-label pre-test: post-test study with blinded outcome assessments, Trials. 12, 62.
- Sharma RR, Pollock K, Hubel A, McKenna D. (2014) Mesenchymal stem or stromal cells: a review of clinical applications and manufacturing practices, Transfusion. 54, 1418-37.
- Lee RH, Pulin AA, Seo MJ, Kota DJ, Ylostalo J, Larson BL, Semprun-Prieto L, Delafontaine P, Prockop DJ. (2009) Intravenous hMSCs improve myocardial infarction in mice because cells embolized in lung are activated to secrete the anti-inflammatory protein TSG-6, Cell Stem Cell. 5, 54-63.
- Zanotti L, Sarukhan A, Dander E, Castor M, Cibella J, Soldani C, Trovato AE, Ploia C, Luca G, Calvitti M, Mancuso F, Arato I, Golemac M, Jonjic N, Biondi A, Calafiore R, Locati M, D’Amico G, Viola A. (2013) Encapsulated mesenchymal stem cells for in vivo immunomodulation, Leukemia. 27, 500-3.
- Timmers L, Lim SK, Arslan F, Armstrong JS, Hoefer IE, Doevendans PA, Piek JJ, El Oakley RM, Choo A, Lee CN, Pasterkamp G, de Kleijn DP. (2007) Reduction of myocardial infarct size by human mesenchymal stem cell conditioned medium, Stem cell research. 1, 129-37.
- Gnecchi M, He H, Liang OD, Melo LG, Morello F, Mu H, Noiseux N, Zhang L, Pratt RE, Ingwall JS, Dzau VJ. (2005) Paracrine action accounts for marked protection of ischemic heart by Akt-modified mesenchymal stem cells, Nature medicine. 11, 367-8.
- Gnecchi M, He H, Noiseux N, Liang OD, Zhang L, Morello F, Mu H, Melo LG, Pratt RE, Ingwall JS, Dzau VJ. (2006) Evidence supporting paracrine hypothesis for Akt-modified mesenchymal stem cell-mediated cardiac protection and functional improvement, The FASEB journal : official publication of the Federation of American Societies for Experimental Biology. 20, 661-9.
- Caplan AI. (2010) What’s in a name? Tissue engineering Part A. 16, 2415-7.
- Caplan Arnold I, Correa D. (2011) The MSC: An Injury Drugstore, Cell Stem Cell. 9, 11-15.
- Caplan AI, Dennis JE. (2006) Mesenchymal stem cells as trophic mediators, Journal of Cellular Biochemistry. 98, 1076-1084.
- Shen C, Lie P, Miao T, Yu M, Lu Q, Feng T, Li J, Zu T, Liu X, Li H. (2015) Conditioned medium from umbilical cord mesenchymal stem cells induces migration and angiogenesis, Mol Med Rep. 12, 20-30.
- Chen L, Xu Y, Zhao J, Zhang Z, Yang R, Xie J, Liu X, Qi S. (2014) Conditioned medium from hypoxic bone marrow-derived mesenchymal stem cells enhances wound healing in mice, PLoS One. 9, e96161.
- Katagiri W, Osugi M, Kinoshita K, Hibi H. (2015) Conditioned Medium From Mesenchymal Stem Cells Enhances Early Bone Regeneration After Maxillary Sinus Floor Elevation in Rabbits, Implant Dent. 24, 657-63.
- van den Berk LC, Jansen BJ, Siebers-Vermeulen KG, Roelofs H, Figdor CG, Adema GJ,Torensma R. (2010) Mesenchymal stem cells respond to TNF but do not produce TNF, J Leukoc Biol. 87, 283-9.
- Tomchuck SL, Zwezdaryk KJ, Coffelt SB, Waterman R, Danka ES, Scandurro AB. (2008) Toll-like receptors on human mesenchymal stem cells drive their migration and immunomodulating responses, Stem Cells. 26, 99-107.
- Shirjang S, Mansoori B, Solali S, Hagh MF, Shamsasenjan K. (2017) Toll-like receptors as a key regulator of mesenchymal stem cell function: An up-to-date review, Cell Immunol. 315, 1-10.
- Kovach TK, Dighe AS, Lobo PI, Cui Q. (2015) Interactions between MSCs and immune cells: implications for bone healing, J Immunol Res. 2015, 752510.
- Kyurkchiev D, Bochev I, Ivanova-Todorov E, Mourdjeva M, Oreshkova T, Belemezova K, Kyurkchiev S. (2014) Secretion of immunoregulatory cytokines by mesenchymal stem cells, World J Stem Cells. 6, 552-70.
- Cassano JM, Schnabel LV, Goodale MB, Fortier LA. (2018) Inflammatory licensed equine MSCs are chondroprotective and exhibit enhanced immunomodulation in an inflammatory environment, Stem Cell Res Ther. 9, 82.
- Xu YX, Chen L, Hou WK, Lin P, Sun L, Sun Y, Dong QY, Liu JB, Fu YL. (2009) Mesenchymal stem cells treated with rat pancreatic extract secrete cytokines that improve the glycometabolism of diabetic rats, Transplant Proc. 41, 1878-84.
- Zheng W, Wang S, Ma D, Tang L, Dua Y, Jin Y. (2009) Loss of proliferation and differentiation capacity of aged human periodontal ligament stem cells and rejuvenation by exposure to the young extrinsic environment, Tissue Eng Part A. 15, 2363-71.
- Ludwig AK, Giebel B. (2012) Exosomes: small vesicles participating in intercellular communication, Int J Biochem Cell Biol. 44, 11-5.
- Yanez-Mo M, Siljander PR, Andreu Z, Zavec AB, Borras FE, Buzas EI, Buzas K, Casal E, Cappello F, Carvalho J, Colas E, Cordeiro-da Silva A, Fais S, Falcon-Perez JM, Ghobrial IM, Giebel B, Gimona M, Graner M, Gursel I, Gursel M, Heegaard NH, Hendrix A, Kierulf P, Kokubun K, Kosanovic M, Kralj-Iglic V, Kramer-Albers EM, Laitinen S, Lasser C, Lener T, Ligeti E, Line, Lipps G, Llorente A, Lotvall J, Mancek-Keber M, Marcilla A, Mittelbrunn M, Nazarenko I, Nolte-‘t Hoen EN, Nyman TA, O’Driscoll L, Olivan M, Oliveira C, Pallinger E, Del Portillo HA, Reventos J, Rigau M, Rohde E, Sammar M, Sanchez-Madrid F, Santarem N, Schallmoser K, Ostenfeld MS, Stoorvogel W, Stukell R Van der Grein SG, Vasconcelos MH, Wauben MH, 27066.
- Raposo G, Stoorvogel W. (2013) Extracellular vesicles: exosomes, microvesicles, and friends, J Cell Biol. 200, 373-83.
- Jeppesen Dk, Hvam ML, Primdahl-Bengtson B, Boysen AT, Whitehead B, Dyrskjot L, Orntoft TF, Howard KA, Ostenfeld MS. (2014) Comparative analysis of discrete exosome fractions obtained by differential centrifugation, J Extracell Vesicles. 3, 25011.
- Taylor DD, Shah S. (2015) Methods of isolating extracellular vesicles impact down-stream analyses of their cargoes, Methods. 87, 3-10.
- Lobb RJ, Becker M, Wen SW, Wong CS, Wiegmans AP, Leimgruber A, Moller A. (2015) Optimized exosome isolation protocol for cell culture supernatant and human plasma, J Extracell Vesicles. 4, 27031.
- Bruno S, Grange C, Deregibus MC, Calogero RA, Saviozzi S, Collino F, Morando L, Busca A, Falda M, Bussolati B, Tetta C, Camussi G. (2009) Mesenchymal stem cell-derived microvesicles protect against acute tubular injury, J Am Soc Nephrol. 20, 1053-67.
- Gatti S, Bruno S, Deregibus MC, Sordi A, Cantaluppi V, Tetta C, Camussi G. (2011) Microvesicles derived from human adult mesenchymal stem cells protect against ischaemia-reperfusion-induced acute and chronic kidney injury, Nephrol Dial Transplant. 26, 1474-83.
- Doeppner TR, Herz J, Gorgens A, Schlechter J, Ludwig AK, Radtke S, de Miroschedji K, Horn PA, Giebel B, Hermann DM. (2015) Extracellular Vesicles Improve Post-Stroke Neuroregeneration and Prevent Postischemic Immunosuppression, Stem Cells Transl Med. 4, 1131-43.
- Potter DR, Miyazawa BY, Gibb SL, Deng X, Togaratti PP, Croze RH, Srivastava AK, Trivedi A, Matthay M, Holcomb JB, Schreiber MA, Pati S. (2018) Mesenchymal stem cell-derived extracellular vesicles attenuate pulmonary vascular permeability and lung injury induced by hemorrhagic shock and trauma, J Trauma Acute Care Surg. 84, 245-256.
- Mellows B, Mitchell R, Antonioli M, Kretz O, Chambers D, Zeuner MT, Denecke B, Musante L, Ramachandra DL, Debacq-Chainiaux F, Holthofer H, Joch B, Ray S, Widera D, David AL, Huber TB, Dengjel J, De Coppi P, Pate K. (2017) Protein and Molecular Characterization of a Clinically Compliant Amniotic Fluid Stem Cell-Derived Extracellular Vesicle Fraction Capable of Accelerating Muscle Regeneration Through Enhancement of Angiogenesis, Stem Cells Dev. 26, 1316-1333.
The rest of this content is restricted - login or subscribe free to access
Thank you for visiting our website. To access this content in full you'll need to login. It's completely free to subscribe, and in less than a minute you can continue reading. If you've already subscribed, great - just login.
Why subscribe? Join our growing community of thousands of industry professionals and gain access to:
- quarterly issues in print and/or digital format
- case studies, whitepapers, webinars and industry-leading content
- breaking news and features
- our extensive online archive of thousands of articles and years of past issues
- ...And it's all free!
Click here to Subscribe today Login here
Related topics
Disease Research, Molecular Biology, Protein, Stem Cells
Related organisations
University of Malaya, University of Reading