Advances in mass spectrometry: unlocking the potential of chemical crosslinking as a structural biology tool
Posted: 16 December 2019 | Professor Albert JR Heck Utrecht (University and the Netherlands Proteomics Centre), Professor Alexander Makarov (Thermo Fisher Scientific), Professor Henning Urlaub (Göttingen University Medical Center), Professor Vicki Wysocki (Ohio State University) | No comments yet
Chemical crosslinking coupled with mass spectrometry (XL-MS) has been used for many years to study protein structure and function. However, recent advances in MS technology, as well as crosslinking reagents and data analysis tools, mean the technique has truly come of age. Here, Albert JR Heck, Alexander Makarov, Henning Urlaub and Vicki Wysocki look at how overcoming XL-MS workflow bottlenecks can unlock the full potential of this approach as a structural biology tool.
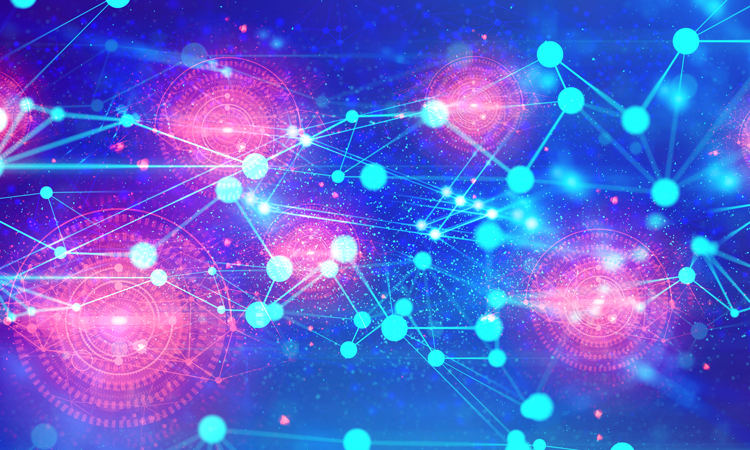
PROTEINS are important in almost all cell-based processes, from cell growth and proliferation through to gene expression and apoptosis. While many proteins act independently, most work in combination. To fully understand how proteins function in biological systems and potentially harness them for the benefit of human health, it is essential to study their structure and the ways in which they interact.
There is a broad range of tools for studying protein–protein interactions (PPIs), including traditional techniques such as X-ray crystallography and nuclear magnetic resonance. However, these approaches often require large quantities of purified sample and do not always enable the analysis of proteins in their native conditions. Cryo-electron microscopy (cryo-EM) has recently emerged as an alternative technique to elucidate PPIs, requiring significantly less sample than traditional techniques. The latest developments in cryo-EM sample preparation, microscope and detector technology, data collection automation and image processing have made it possible to reproducibly achieve near-atomic levels of resolution. As cryo-EM uncovers more challenging protein complexes and scientists investigate PPIs in their native environment, the need for complementary methods has never been greater.
A powerful tool for structural biology
XL-MS can probe the nature of PPIs by physically connecting proteins to each other as they interact using chemical crosslinking reagents. By digesting crosslinked proteins into peptides and characterising these fragments using MS, the structure of the interacting protein complex can be pieced together. Furthermore, because each spacer arm is of a fixed length and binds with specific amino acid residues, scientists are not only able to identify which proteins interact with each other, but also to pinpoint precisely where each protein makes that connection.
MS-based structural biology techniques promise to open a wealth of opportunities for human health”
“Chemical crosslinking is a powerful tool for protein structural analysis and it complements alternative techniques such as X-ray crystallography and cryo-EM very well,” explains Professor Henning Urlaub, bioanalytical MS group leader at the Max‑Planck Institute in Göttingen. “The resolution offered by MS allows you to study much smaller proteins than is possible using cryo-EM and the information obtained can be used to identify new protein interactions and complexes and understand how they function.
“By crosslinking kinases, for example, with their substrates you can probe how they interact and then see how these interactions change upon phosphorylation. Once you understand these interactions more fully, you can then start to design better drugs to interact and interfere with them.”
Recently, there have been considerable advances across the three fundamental components of XL‑MS techniques: chemical crosslinking reagents, MS detection and informatics for data analysis.
Generating powerful insight with MS-cleavable crosslinkers
Crosslinking workflows involves joining neighbouring proteins using chemical crosslinking reagents. These reagents consist of two functional groups joined by a spacer arm. Traditionally, the spacer arms employed in most crosslinking reagents remained intact during MS fragmentation. However, analysing the results of XL-MS studies using non-cleavable crosslinkers was often highly challenging due to the difficulties associated with distinguishing between crosslinked and non-crosslinked peptides.
The recent development of MS-cleavable crosslinkers designed to break in the gas phase during MS2 analysis has significantly simplified data analysis. Following collision-induced separation, MS-cleavable crosslinkers, such as disuccinimidyl sulfoxide (DSSO) and disuccinimidyl dibutyric urea (DSBU), generate two linear peptides that contain parts of the crosslinking reagent, differentiating them from the non-crosslinked peptide (Figure 1). With knowledge of the MS signature of the cleavable crosslinker, peptides can be sequenced and identified by MS3 analysis, accelerating data processing.
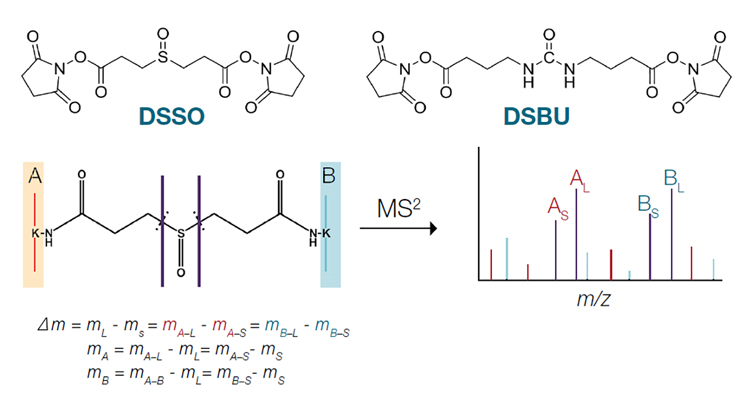
Figure 1: Fragmentation of MS-cleavable crosslinkers by MS2
Confident peptide sequencing using maximum performance hybrid MS instruments
The next stage in XL-MS workflows involves analysing crosslinked peptides by MS. Crosslinked peptides are present at levels several thousand, or even million, times less abundant than unmodified peptides, making analysis extremely challenging. Over the past decade, XL-MS techniques have benefitted enormously from advances in MS, with the greater availability of sophisticated fragmentation techniques being particularly important.
“XL-MS experiments rely on knowledge of both crosslinked peptides,” explains Albert Heck, professor of Biomolecular MS and Proteomics at Utrecht University. “No matter how well you sequence one peptide, if you can’t identify the other because it is too small or does not fragment favourably, then unfortunately your analysis isn’t worth anything.”
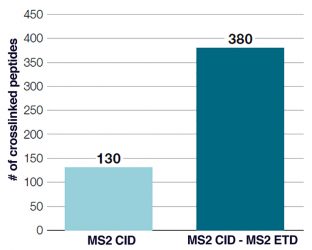
Figure 2: Improved coverage of crosslinked peptides obtained using MS2 CID – MS2 ETD acquisition
Traditional MS2 fragmentation techniques, such as collision-induced dissociation (CID) and higher energy collisional dissociation (HCD), often generate unequal peptide fragment ions, making peptide identification challenging. One way to improve sequence coverage is to use multiple fragmentation techniques in combination (Figure 2).1,2 Advanced acquisition methods such as electron transfer/higher energy collision dissociation (EThcD), which combine electron transfer dissociation (ETD) with HCD in one workflow, can maximise the amount of useful sequence information that can be obtained from XL-MS studies.3,4
“Modern hybrid mass spectrometers are increasingly sophisticated and allow you to combine a wide range of fragmentation techniques with different collision energies – they are almost like an entire MS lab in a single instrument,” Heck adds. “Systems that combine quadrupole, linear ion trap and Orbitrap analysers allow you to rapidly undertake, for example, CID followed by ETD, giving you the flexibility to sequence fragment ions with much greater confidence.”
The wider adoption of XL-MS has enabled the establishment of templates that allow users to quickly and conveniently select the fragmentation schemes they need. By making these techniques accessible to all users, rather than just specialists, more scientists can benefit from the power of the latest MS instruments.
Building the complete picture with powerful data analysis
To make sense of the large amounts of data generated from XL-MS experiments, powerful informatics solutions are required. However, a lack of commercially available software designed specifically for XL-MS data has often required teams to develop their own software, which can be resource intensive.
“The considerable advances in both MS technologies and crosslinking reagents that have been made over the past decade have meant that data analysis has now become one of the biggest workflow bottlenecks,” explains Dr Alexander Makarov, Director of Global Research for life sciences MS at Thermo Fisher Scientific. “Fortunately, recent years have seen improvements in the commercial informatics solutions available. These have led to improvements in the identification of false positives and negatives, as well as in statistical processing.”
XL-MS allows scientists to identify the proteins involved in previously unknown PPIs”
One algorithm that has been developed to capitalise on the analytical power of cleavable crosslinkers is XlinkX,5 which was developed by Utrecht University’s Heck group. This takes advantage of the unique signature ions that are generated through crosslinker cleavage. These signals give the masses of the crosslinked peptides directly, eliminating the need to search all proteome combinations by trial-and-error and significantly reducing analysis time. “To get a comprehensive picture of PPIs, you have to gather information using a range of structural biology tools,” says Makarov. “Currently, combining information from crystallography, electron microscopy and other sources is a manual process that is often very time consuming and requires a large amount of specialist expertise. There’s a huge opportunity for artificial intelligence to help scientists stitch together this data in a more straightforward way.”
Using XL-MS alongside other structural biology techniques
XL-MS is considered a complementary tool for the wide range of techniques already available to protein scientists. In the increasingly important field of cryotomography, for example, XL-MS allows scientists to identify the proteins involved in previously unknown PPIs, as such insight cannot be achieved by cryo-EM alone. Additionally, crosslinking workflows can be easily performed by laboratories already set up for MS protein analysis.
This is a view that is shared by Vicki Wysocki, professor of chemistry and biochemistry at Ohio State University. Her team uses a wide range of MS instruments to study the structure and interactions of protein complexes to advance human health. Ongoing projects include those designed to better understand protein-RNA complexes, such as those associated with HIV, as well as analysing biomedically important natural and designed protein complexes.6
“The analytical power and flexibility of these instruments means we use them in a lot of different ways,” Wysocki explains. “Many of our studies involve electrospray of intact complexes of proteins with other proteins or with RNA or DNA, and dissociation by collision with a surface. Given the large size of these complexes, we use ultra-high mass range (UHMR) Orbitrap mass analyser instruments to measure the high mass-to-charge (m/z) ratios, with fragment ions sometimes in excess of 30,000 m/z.”7
While traditional crosslinking techniques involve the digestion of crosslinked proteins into smaller peptide fragments, Wysocki’s team intend to combine selective chemical crosslinking techniques with UHMR native MS to study intact crosslinked proteins and protein complexes. This may be of particular relevance for cryo-EM research, where crosslinkers are used to stabilise protein complexes prior to sample vitrification.
Conclusion
With advances in the power and flexibility of hybrid MS technologies and improvements in chemical linkers and informatics solutions, XL-MS is coming of age. With the latest developments in analyser technology also expanding the capabilities of other structural biology tools, MS-based structural biology techniques promise to open a wealth of opportunities for human health.
About the authors
Professor Vicki Wysocki received her PhD from Purdue University in 1987, under Professor Graham Cooks. At the Ohio State University she is an Ohio Eminent Scholar and Director of the Campus Chemical Instrument Center. She is an associate editor for the ACS journal Analytical Chemistry and is Past President of ASMS.
Professor Albert JR Heck is a professor in Biomolecular Mass Spectrometry and Proteomics at Utrecht University and scientific director of the Netherlands Proteomics Centre. Heck’s group emphasises on the development and applications of advanced mass spectrometrybased proteomics technologies. Heck’s lab has been instrumental in introducing novel MS instrumentation for native and top-down MS.
Professor Alexander Makarov obtained his PhD at Moscow Engineering Physics Institute in 1993. His work on the Orbitrap mass analyser led to its commercial launch in 2005. He is currently a Chair at Utrecht University (Netherlands) and holds the position of Director of Research in Thermo Fisher Scientific.
After studying biochemistry, Professor Henning Urlaub obtained his PhD at the FU Berlin. Following post-doctoral work at Marburg University and at the Max-Planck Institute for Biophysical Chemistry (MPIbpc) in Göttingen, Germany, he became an independent research group leader at MPIbpc and later on a full professor at Göttingen University Medical Center.
References
- Liu F, Rijkers DT, Post H, Heck AJ. Proteome-wide profiling of protein assemblies by cross-linking mass spectrometry. Nat Methods. 2015; 12(12): 1179–1184.
- Bomgarden R, Raja E, Etienne C, Liu F, Heck AJ, Mueller M, et al. Optimization of crosslinked peptide analysis on an Orbitrap Fusion Lumos mass spectrometer [poster]. ASMS 2016.
- Frese CK, Altelaar AF, van den Toorn H, Nolting D, Griep-Raming J, Heck AJ, et al. Toward full peptide sequence coverage by dual fragmentation combining electron-transfer and higher-energy collision dissociation tandem mass spectrometry. Anal Chem. 2012; 84(22): 9668–9673.
- Frese CK, Zhou H, Taus T, Altelaar AF, Mechtler K, Heck AJ, et al. Unambiguous phosphosite localization using electron-transfer/higher-energy collision dissociation (EThcD). J Proteome Res. 2013; 12(3): 1520–1525.
- Liu F, Lössl P, Scheltema R, Viner R, Heck AJR. Optimized fragmentation schemes and data analysis strategies for proteome-wide cross-link identification. Nature Commun. 2017; 8: 15473.
- Chen Z, Boyken S, Jia M, Busch F, Flores-Solis D, Bick MJ, et al. Programmable design of orthogonal protein heterodimers. Nature. 2019; 565: 106–111.
- van de Waterbeemd M, Fort KL, Boll D, Reinhardt-Szyba M, Routh A, Makarov A, et al. High-fidelity mass analysis unveils heterogeneity in intact ribosomal particles. Nat Methods. 2017; 14(3): 283–286.
Related topics
Crystallography, Mass Spectrometry, Protein, Research & Development, Targets, X-ray Crystallography