The genetic modifier approach: identifying the right target for rare diseases
Posted: 21 June 2023 | Dr Vincent Blomen (Scenic Biotech) | No comments yet
In this article, Dr Vincent Blomen, Senior Director of Target Discovery at Scenic Biotech, takes us through the realm of rare diseases. In the case of most of these diseases, a singular genetic anomaly often reigns supreme, yet its impact on patients can be vastly divergent. Enter modifier genes, the key to unraveling this variability and potentially mitigating or halting disease development altogether. However, identifying these elusive genes poses a formidable challenge. Nonetheless, unlocking the potential for systematic discovery of these uncharted targets presents a golden opportunity for pioneering novel therapies.
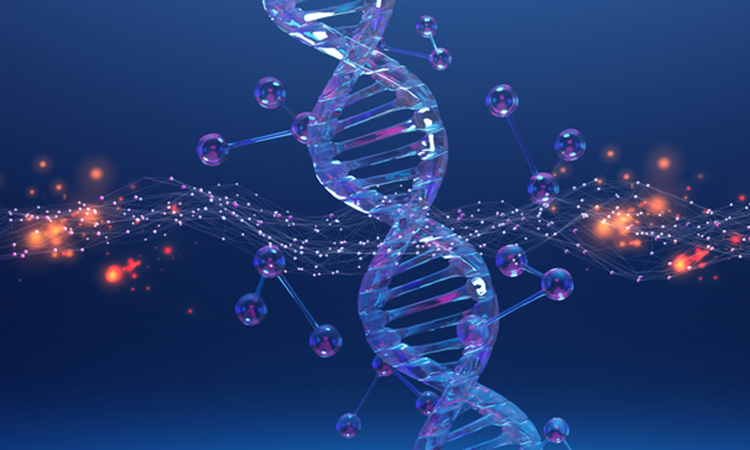
Most rare diseases are caused by a single gene defect, but severity can vary considerably among patients. Modifier genes can help explain that variability and can alter or even prevent disease onset and progression, making them appealing therapeutic targets. However, the identification of these genes is challenging. Enabling the systematic discovery of these largely uncharted targets can be a valuable opportunity for development of novel therapies.
Genetic-based therapeutics hold significant promise in the clinic
Despite the growing number of genes linked to human disease, the proportion of genes targeted by approved therapies remains low. A significant challenge in developing novel therapies is the limited understanding of how the target gene influences the underlying disease biology, which contributes to the considerable attrition observed in investigative therapies.1 With novel emerging drug modalities further expanding the druggable genome, connecting the right target genes to a disease will only become more relevant. Strikingly, a strong genetic link between target and disease biology is emerging as a predictor for success in clinical trials.2,3 This is exemplified by drugs that target disease-specific genes or genetically distinct patient subsets which are more likely to succeed in demonstrating efficacy in clinical development.4 For the treatment of rare genetic disorders especially, drugs with genetically supported targets are more than twice as likely to be approved5, thereby indicating genetics and genomics can empower companies to develop better drugs.
Leveraging genetic evidence to develop new therapeutics has been successful in the past. An example is Vertex Pharmaceuticals’ approved treatment for cystic fibrosis (Kalydeco)6, which counteracts the outcome of a specific mutation thereby stabilising the defective CTFR protein, and restoring proper pulmonary function. This is one of many examples where genetic information was critical in generating a clinically effective and efficient compound. As most inherited diseases are caused by gene mutations that lead to a defect or loss of function, the benefit of using genetics to find new drug targets is compelling. Nevertheless, directly targeting a protein encoded by a disease-causing variant is rarely simple.
Genetic modifiers: the safeguard for genetic disorders
This is where genetic modifiers come into play. Genetic modifiers are genes that can influence the phenotypic outcome of a disease-causing gene variant, thereby either exacerbating or ameliorating the severity of the disease.7.This results in a diverse range of symptoms among patients. Especially in rare disorders, individuals with identical pathogenic mutations may present with different clinical symptoms. For example, Gaucher disease, an autosomal recessive lysosomal storage disorder caused by mutations in the GBA1 gene, manifests in different phenotypes even within families that have similar genotypes.8 Genetic modifiers that suppress the effects of a disease-causing gene, genetic suppressors, can protect carriers from the disease. They may dampen and, in some instances, even fully overcome monogenic disease traits. A human genetics study involving over half a million genomes from healthy people notably identified a small number of individuals that carried fully penetrant disease mutations, yet did not exhibit any clinical symptoms of the disease.9 Such insights highlight the power of genetic modifiers in inherited conditions and their therapeutic potential. Nevertheless, their identification is a challenging task and thus remains an underexplored area of study. However, dvancements have led to renewed interest in genetic modifiers and their prospects in the development of novel therapeutics for rare diseases.
Identifying genetic modifiers as new therapeutic targets
How can we tackle the elusive search for genetic modifiers?
A highly complementary approach to uncover genetic modifiers is by using functional genomics in human cells.
Methods such as human population genetics, model organisms and functional genomics have been effectively employed for that purpose.10 Furthermore, improved access to patient whole genome sequencing data and recent progress in genome editing are now enabling both observational and experimental approaches to identify modifier genes, notably for rare diseases.
In the human genetics field, genetic modifiers have been successfully identified through family linkage analyses11, genome-wide association studies (GWAS)12, and using whole-genome (or exome) sequencing.13 While the increasing availability of genome sequencing will facilitate the identification of modifier genes in the future, there are a few inherent challenges. For one, these approaches are reliant on the inclusion of individuals with modifier variants. However, resilient individuals are infrequent in the general population9 and in more targeted studies examining a specific disease, patients with a modified course of disease may elude inclusion due to effective suppression or a compound phenotype. The modest sample sizes which are typical in rare disease research make it inherenttly challenging to obtain sufficient power to confidently associate gene variants to phenotypes. Lastly, interpreting the functional relevance of gene variants associated with phenotypic outcome is not straightforward. Nonetheless, genomics-based identification will only increase in relevance for the identification of modifier genes as genome sequencing becomes more prolific and the analysis toolbox expands.
Studies in model organisms have long observed that the genetic background is a major determinant of phenotypic outcome.14 In particular in baker’s yeast, researchers could systematically identify gene-gene interactions, including genetic suppressors.15. Access to complex libraries of mutant strains yielded significant insights into genetic suppressor interactions which suggests similar genetic connections are relevant to human phenotypes.16 Since directly translating genetic interactions from simple model organisms into human traits or diseases is not clear-cut, more complex model organisms such as flies, worms, zebrafish, mice, and dogs have been used to identify modifier genes. For example, a study conducted in dogs with Duchenne’s Muscular Dystrophy (DMD) singled out two specimen with a mild form of the disease. Using a combination of sequencing-based approaches and experimental validation in zebrafish, researchers showed that increased jagged1 activity could suppress the disease in dogs.17 Nevertheless, the use of complex model organisms for the systematic identification of genetic modifiers is challenging due to longer generation times and high costs, and is therefore most frequently reserved for reverse genetic approaches that examine a small number of candidate modifiers or for characterising a few healthy carriers.
A highly complementary approach to uncover genetic modifiers is by using functional genomics in human cells. Innovative functional genomics platforms have been set up by companies such as Maze Therapeutics, Scenic Biotech, generating interesting results with clinical studies in process.
Using functional genomics to find genetic modifiers
Functional genomic approaches can explore the whole genome of mammalian cells to not only identify modifier genes but also establish their relation to a relevant cellular disease phenotype. Many rare metabolic disorders feature disrupted elementary processes, the consequences of which can frequently measured in cells carrying disease mutations. Thanks to high-throughput genome editing approaches, activating or inactivating mutations can be introduced on a genome-wide scale and their effects on measurable disease traits can easily be analysed. This in contrast to population genetics studies which rely on the presence of rare individuals carrying modifier variants. Although there are limitations to modeling diseases in cell systems, the use of human cells increases the likelihood of identifying robust and species-relevant genetic interactions. Hence, functional genomic approaches offer a highly complementary path toward the rapid identification of modifier genes, which has become more and more valuable in both academic and industry settings.
Maze Therapeutics, for instance, has developed the Compass platform which combines functional genomics with human genetic data in order to develop new precision medicines for genetically validated drug targets. One of their programs focuses on amyotrophic lateral sclerosis (ALS), a fatal neurodegenerative disorder that translates into progressive muscle weakness and eventually death within 2-5 years. The knowledge that in some ALS cases patients live well beyond this prognosis has led Maze Therapeutics to identify a genetic modifier that impacts the severity of the disease. Inhibition of the modifier was found to restrict the toxicity of the protein TDP-43, which participates in pathologic aggregates observed in ALS patients.18 The company is now attempting to develop a microRNA therapy that targets the gene ATXN2 and has already shown that loss of this gene has a positive effect on survival in preclinical models.
Pioneering research on genetic modifiers is also performed at Scenic Biotech to develop a new generation of therapeutics. Their proprietary Cell-Seq platform uses human haploid cells (harboring only one copy of each chromosome) which are amenable to random mutagenesis.19,20 Mutations are introduced across the genome of these cells through gene-trap mutagenesis and the resulting phenotypes can be monitored with fluorescent markers in both wildtype cells and cells with an engineered disease mutations.21 The gene trap mutations introduce a molecular tag into the genome to pinpoint the genetic modifiers that affect the disease phenotype. This approach can be applied to a wide range of disorders to search systematically for genetic modifiers within the genome. This concept has led to a collaboration with Genentech, a member of the Roche Group, under which Scenic uses its platform and insight to discover novel therapeutics that target genetic modifiers.
Scenic Biotech’s therapeutic focus now is on unveiling genetic modifiers for rare diseases, with Niemann-Pick Type C (NPC) and Barth Syndrome as some of the diseases under investigation. Barth Syndrome is an inherited mitochondrial disorder that arises from mutations in the gene encoding for Tafazzin. Patients with Barth’s syndrome have a reduced life expectancy and there are currently no effective treatments available other than symptomatic care. Niemann-Pick Type C is a rare lipid storage disorder caused by a mutation in the NPC1 gene, which results in disruption of lipid metabolism. NPC is mainly treated with miglustat, a glucosylceramide synthase (GCS) inhibitor that delays the appearance of neurological symptoms.22 However, this therapy is only approved for NPC in Europe and the illness provokes many other symptoms, with a current high unmet medical need as a result. In February 2023, Scenic Biotech announced a Cooperative Research and Development Agreement (CRADA) with the National Institutes of Health (NIH) to support the identification of genetic modifiers for this severe indication with patient genomic and clinical data to further complement and reinforce Scenic’s proprietary target discovery platform. Genetic modifier-based approaches could eventually provide a pharmacological recourse to not only delay but also reverse the damage caused by the disease on both a neurological and physiological level. Identifying and targeting genetic modifiers therefore presents as a promising path towards such treatment options.
Genetic modifiers: the path ahead
The therapeutic potential of genetic modifiers is gradually emerging for an array of indications, as their influence on inherited disease phenotypes becomes more evident and their identification more attainable. Indeed, targeting genes that impact disease representation, rather than the disease-causing genes, constitutes a particularly novel therapeutic approach that companies like Scenic Biotech and Maze Therapeutics are now equipped to employ in their search for new drug targets. Their cutting-edge platforms should allow them to overcome the hurdles that uncovering genetic modifiers implies to develop breakthrough medicines. The coming years will be critical in conquering the genetic modifiers field and demonstrating this area of research is a strong therapeutic avenue for the treatment of diseases with high unmet medical needs.
Author’s bio:
Dr Vincent Blomen
Vincent is the Senior Director of Target Discovery at Scenic Biotech. He completed his PhD at Utrecht University, where he worked in the lab of one of Scenic’s founders. During his PhD, he contributed to the development of a platform to identify genetic interactions, which is now used at Scenic to discover modifier genes. In his current role, Vincent oversees the discovery efforts of new drug targets using Scenic’s proprietary platform.
References:
- Finan C, Gaulton A, Kruger FA, Lumbers RT, Shah T, Engmann J, Galver L, Kelley R, Karlsson A, Santos R, Overington JP, Hingorani AD, Casas JP. The druggable genome and support for target identification and validation in drug development. Sci Transl Med. 2017 Mar 29;9(383):eaag1166. Doi: 10.1126/scitranslmed.aag1166. PMID: 28356508; PMCID: PMC6321762.
- Razuvayevskaya O, Lopez I, Dunham I, Ochoa D. Why Clinical Trials Stop: The Role of Genetics. medRxiv; 2023. DOI: 10.1101/2023.02.07.23285407.
- Ochoa D, Karim M, Ghoussaini M, Hulcoop DG, McDonagh EM, Dunham I. Human genetics evidence supports two-thirds of the 2021 FDA-approved drugs. Nat Rev Drug Discov. 2022 Aug;21(8):551. Doi: 10.1038/d41573-022-00120-3. PMID: 35804044.
- Chi Heem Wong, Kien Wei Siah, Andrew W Lo, Estimation of clinical trial success rates and related parameters, Biostatistics, Volume 20, Issue 2, April 2019, Pages 273–286,
- King EA, Davis JW, Degner JF. Are drug targets with genetic support twice as likely to be approved? Revised estimates of the impact of genetic support for drug mechanisms on the probability of drug approval. PloS Genet. 2019 Dec 12;15(12):e1008489. Doi: 10.1371/journal.pgen.1008489. PMID: 31830040; PMCID: PMC6907751.
- Davis PB, Yasothan U, Kirkpatrick P. Ivacaftor. Nat Rev Drug Discov. 2012 Apr 30;11(5):349-50. Doi: 10.1038/nrd3723. PMID: 22543461.
- Riordan JD, Nadeau JH. From Peas to Disease: Modifier Genes, Network Resilience, and the Genetics of Health. Am J Hum Genet. 2017 Aug 3;101(2):177-191. Doi: 10.1016/j.ajhg.2017.06.004. PMID: 28777930; PMCID: PMC5544383.
- Davidson, BA, Hassan, S, Garcia, EJ, Tayebi, N, Sidransky, E. Exploring genetic modifiers of Gaucher disease: The next horizon. Human Mutation. 2018; 39: 1739– 1751.
- Chen R, Shi L, Hakenberg J, Naughton B, Sklar P, Zhang J, Zhou H, Tian L, Prakash O, Lemire M, Sleiman P, Cheng WY, Chen W, Shah H, Shen Y, Fromer M, Omberg L, Deardorff MA, Zackai E, Bobe JR, Levin E, Hudson TJ, Groop L, Wang J, Hakonarson H, Wojcicki A, Diaz GA, Edelmann L, Schadt EE, Friend SH. Analysis of 589,306 genomes identifies individuals resilient to severe Mendelian childhood diseases. Nat Biotechnol. 2016 May;34(5):531-8. Doi: 10.1038/nbt.3514. Epub 2016 Apr 11. PMID: 27065010.
- Rahit KMTH, Tarailo-Graovac M. Genetic Modifiers and Rare Mendelian Disease. Genes (Basel). 2020 Feb 25;11(3):239. Doi: 10.3390/genes11030239. PMID: 32106447; PMCID: PMC7140819.
- Mouton JM, van der Merwe L, Goosen A, Revera M, Brink PA, Moolman-Smook JC, Kinnear C. MYBPH acts as modifier of cardiac hypertrophy in hypertrophic cardiomyopathy (HCM) patients. Hum Genet. 2016 May;135(5):477-483. Doi: 10.1007/s00439-016-1649-7. Epub 2016 Mar 11. PMID: 26969327.
- Corvol H, Blackman SM, Boëlle PY, Gallins PJ, Pace RG, Stonebraker JR, Accurso FJ, Clement A, Collaco JM, Dang H, Dang AT, Franca A, Gong J, Guillot L, Keenan K, Li W, Lin F, Patrone MV, Raraigh KS, Sun L, Zhou YH, O’Neal WK, Sontag MK, Levy H, Durie PR, Rommens JM, Drumm ML, Wright FA, Strug LJ, Cutting GR, Knowles MR. Genome-wide association meta-analysis identifies five modifier loci of lung disease severity in cystic fibrosis. Nat Commun. 2015 Sep 29;6:8382. Doi: 10.1038/ncomms9382. PMID: 26417704; PMCID: PMC4589222.
- Wilk MA, Braun AT, Farrell PM, Laxova A, Brown DM, Holt JM, Birch CL, Sosonkina N, Wilk BM, Worthey EA. Applying whole-genome sequencing in relation to phenotype and outcomes in siblings with cystic fibrosis. Cold Spring Harb Mol Case Stud. 2020 Feb 3;6(1):a004531. Doi: 10.1101/mcs.a004531. PMID: 32014855; PMCID: PMC6996517.
- Olguín V, Durán A, Las Heras M, Rubilar JC, Cubillos FA, Olguín P, Klein AD. Genetic Background Matters: Population-Based Studies in Model Organisms for Translational Research. Int J Mol Sci. 2022 Jul 8;23(14):7570. Doi: 10.3390/ijms23147570. PMID: 35886916; PMCID: PMC9316598.
- Tong AH, Lesage G, Bader GD, Ding H, Xu H, Xin X, Young J, Berriz GF, Brost RL, Chang M, Chen Y, Cheng X, Chua G, Friesen H, Goldberg DS, Haynes J, Humphries C, He G, Hussein S, Ke L, Krogan N, Li Z, Levinson JN, Lu H, Ménard P, Munyana C, Parsons AB, Ryan O, Tonikian R, Roberts T, Sdicu AM, Shapiro J, Sheikh B, Suter B, Wong SL, Zhang LV, Zhu H, Burd CG, Munro S, Sander C, Rine J, Greenblatt J, Peter M, Bretscher A, Bell G, Roth FP, Brown GW, Andrews B, Bussey H, Boone C. Global mapping of the yeast genetic interaction network. Science. 2004 Feb 6;303(5659):808-13. Doi: 10.1126/science.1091317. PMID: 14764870.
- van Leeuwen J, Pons C, Mellor JC, Yamaguchi TN, Friesen H, Koschwanez J, Ušaj MM, Pechlaner M, Takar M, Ušaj M, VanderSluis B, Andrusiak K, Bansal P, Baryshnikova A, Boone CE, Cao J, Cote A, Gebbia M, Horecka G, Horecka I, Kuzmin E, Legro N, Liang W, van Lieshout N, McNee M, San Luis BJ, Shaeri F, Shuteriqi E, Sun S, Yang L, Youn JY, Yuen M, Costanzo M, Gingras AC, Aloy P, Oostenbrink C, Murray A, Graham TR, Myers CL, Andrews BJ, Roth FP, Boone C. Exploring genetic suppression interactions on a global scale. Science. 2016 Nov 4;354(6312):aag0839. doi: 10.1126/science.aag0839. PMID: 27811238; PMCID: PMC5562937.
- Vieira NM, Elvers I, Alexander MS, Moreira YB, Eran A, Gomes JP, Marshall JL, Karlsson EK, Verjovski-Almeida S, Lindblad-Toh K, Kunkel LM, Zatz M. Jagged 1 Rescues the Duchenne Muscular Dystrophy Phenotype. Cell. 2015 Nov 19;163(5):1204-1213. doi: 10.1016/j.cell.2015.10.049. Epub 2015 Nov 12. PMID: 26582133; PMCID: PMC4668935.
- Ma XR, Prudencio M, Koike Y, Vatsavayai SC, Kim G, Harbinski F, Briner A, Rodriguez CM, Guo C, Akiyama T, Schmidt HB, Cummings BB, Wyatt DW, Kurylo K, Miller G, Mekhoubad S, Sallee N, Mekonnen G, Ganser L, Rubien JD, Jansen-West K, Cook CN, Pickles S, Oskarsson B, Graff-Radford NR, Boeve BF, Knopman DS, Petersen RC, Dickson DW, Shorter J, Myong S, Green EM, Seeley WW, Petrucelli L, Gitler AD. TDP-43 represses cryptic exon inclusion in the FTD-ALS gene UNC13A. Nature. 2022 Mar;603(7899):124-130. doi: 10.1038/s41586-022-04424-7. Epub 2022 Feb 23. PMID: 35197626; PMCID: PMC8891019.
- Carette JE, Guimaraes CP, Varadarajan M, Park AS, Wuethrich I, Godarova A, Kotecki M, Cochran BH, Spooner E, Ploegh HL, Brummelkamp TR. Haploid genetic screens in human cells identify host factors used by pathogens. Science. 2009 Nov 27;326(5957):1231-5. doi: 10.1126/science.1178955. PMID: 19965467.
- Park, D., Han, C., Elliott, M. et al.Continued clearance of apoptotic cells critically depends on the phagocyte Ucp2 protein. Nature 477, 220–224 (2011). https://doi.org/10.1038/nature10340
- Brockmann M, Blomen VA, Nieuwenhuis J, Stickel E, Raaben M, Bleijerveld OB, Altelaar AFM, Jae LT, Brummelkamp TR. Genetic wiring maps of single-cell protein states reveal an off-switch for GPCR signalling. Nature. 2017 Jun 8;546(7657):307-311. doi: 10.1038/nature22376. Epub 2017 May 31. PMID: 28562590.
- Pineda M, Walterfang M, Patterson MC. Miglustat in Niemann-Pick disease type C patients: a review. Orphanet J Rare Dis. 2018 Aug 15;13(1):140. doi: 10.1186/s13023-018-0844-0. PMID: 30111334; PMCID: PMC6094874.
Related topics
Animal Models, Disease Research, Genetic Analysis, Genomics, microRNA, Proteogenomics, RNAs
Related conditions
Amyotrophic Lateral Sclerosis (ALS), Barth syndrome, Duchenne’s Muscular Dystrophy (DMD), Niemann-Pick Type C (NPC)
Related organisations
Maze Therapeutics, National Institutes of Health (NIH), Scenic Biotech