Epigenetic editing: the next generation of genetic medicine
Posted: 7 July 2023 | Dr Blythe Sather | No comments yet
Dr Blythe Sather at Tune Therapeutics reveals why epigenetic editing offers benefits above and beyond those of gene editing, potentially bridging the gaps to breakthroughs in the clinic.
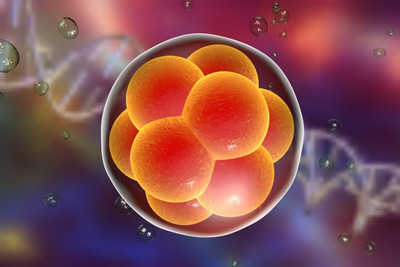
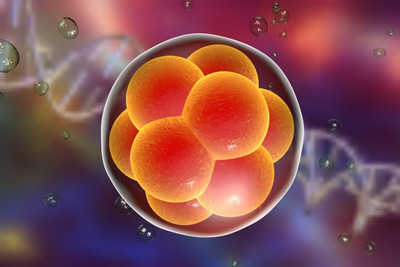
When targeted gene editing became a reality more than ten years ago, many people hoped for a revolutionary tsunami of new medical treatments. In the wake of the Human Genome Project, the promise of precision medicine is marching forward, yet the clinic has experienced a mere trickle of new therapeutics so far. The reasons for this are multifaceted, including concerns over the safety of directly altering DNA sequences and subsequent regulatory restrictions that have arisen as a result. The slow translational adoption makes it clear that scientists need new tactics to activate the latent potential of genetic medicine.
Epigenetic editing is the innovative new approach that the field has long awaited. This pioneering technology adjusts gene transcription through the epigenome, directly targeting the root of dysregulation while leaving the primary DNA sequence intact. Here we explore the how and why of epigenetic editing — how it works, its advantages over alternative methods, and the exciting clinical potential it holds.
What is epigenetic editing?
The epigenome (meaning ‘above the genome’) is a system of reversible marks regulating how the DNA is read, translated and used. DNA encodes the basic building blocks of life and the epigenome controls which blocks are accessible for active use. The molecular machinery of the epigenetic system can selectively package specific regions of DNA away, making them inaccessible and less active. The system can also unwind the DNA to expose it for active use.
Epigenetic editing, also called genetic tuning, actively manipulates epigenetic machinery to influence transcription. Orchestrating the naturally-occurring conductors of gene expression can shift the functional states of cells and rebalance gene expression.
This modality is entirely distinct from the ‘molecular scissors’ approach traditionally associated with gene editing. While epigenetic editing and nuclease-based gene editing use similar machinery to direct their effectors to the DNA, epigenetic editing is an entirely ‘no-cut’ process. Instead of slicing into the DNA like a nuclease, epigenetic editing alters the epigenomic marks adorning the DNA, leaving the base code untouched.
This mechanism gives epigenetic editing unprecedented control of gene expression, allowing individuals to fine-tune gene output. This precision has powerful therapeutic implications because shifting the epigenetic signature of a cell changes its transcriptional profile and, ultimately, its biological activity.
What are the advantages of epigenetic editing?
Epigenetic editing is a bold methodology born from the need to expand the potential of genetic medicine. But what sets it apart from other techniques? The method is unique because it leaves the native DNA intact, has powerful multiplexing capabilities, and thus offers tremendous flexibility in gene output. These benefits expand the potential range of therapeutic targets available to precision medicine beyond those of other therapeutic approaches.
Avoiding DNA damage
As described, epigenetic editing is a ‘no-cut’ process. This avoids potentially harmful DNA damage such as chromosomal translocations,1 which are associated with several tumour types. Additionally, because epigenetic editing does not directly alter the genetic source code, on the rare occasion that off-target effects occur, they are generally benign. Put another way, epigenetic editing shares the specificity of nuclease-based genetic editing while minimising the risks associated with directly cutting into the genome.
Multiplexing
Another marked advantage of epigenetic editing is the potential for multiplexing, ie, targeting several genes simultaneously. Many disease states are caused by a web of complex interactions rather than single-gene mutations. As a result, the solution to these pathologies requires a multigenic approach. While in theory gene editing can cut several locations simultaneously, in practice, creating multiple double-strand DNA breaks can potentially cause genomic instability. Epigenetic editing eliminates this risk by leaving the DNA intact. This gives epigenetic editing the unique ability to treat complex multigenic diseases and opens the door to enhancing cell and regenerative therapies, which require the manipulation of multiple genes for optimal results.
Nuanced and highly controlled adjustments
Epigenetic editing goes beyond the binary on-off switch state achieved by gene editing and can instead create a dynamic range of expression levels. The field of epigenetic editing includes a growing suite of tools that can deliver precise gene dosages with impressive control. This is particularly critical in cases where over- or under-expression of a gene can be toxic, as in haploinsufficiency syndromes.2
Additionally, the epigenetic editing toolkit allows for precise temporal regulation. The innate systems of epigenetic memory allow for the creation of enduring, heritable alterations or brief temporary changes in transcription. This level of control is not achievable with gene editing and is a severe limitation in applications such as T-cell engineering, where precise epigenetic remodelling during differentiation3 fundamentally shapes the phenotype and function of the cell.
The epigenetic editing toolbox continues to grow increasingly more sophisticated with tools that facilitate greater precision. For example, chemical modifications to the guide RNA — responsible for directing the epigenetic machinery to the region of interest — can fine-tune the intensity and stability of transcriptional changes. Furthermore, scientists are engineering diverse targeting systems such as modified CRISPR-Cas complexes, zinc fingers and transcription activator-like effectors to tackle epigenetic targets with enhanced specificity and durability. As we discover more about the molecular players regulating transcription, new options will emerge, enabling finer control over the dosage and duration of epigenetic changes.
Epigenetic editing in the clinic
With tightly controlled gene regulation and unparalleled multiplexing capabilities, epigenetic editing has profound clinical potential. So, where does epigenetic editing currently stand and how will it transition into the clinic?
Epigenetic editing has a strong foundation in the lab, and ample data has shown safety and effectiveness in non-clinical studies. For example, researchers used it to induce transcriptional silencing in the liver of mice,4 resulting in an enduring reduction in cholesterol levels. In patient-derived induced pluripotent stem cells with fragile X syndrome, epigenetic editing successfully reversed hypermethylation,5 effectively restoring healthy expression. Beyond targeted expression changes, epigenetic editing can also perform high-throughput screens6 to identify functional regulatory elements, which may be critical for effectively engineering stem and immune cells as well as programming tissue regeneration. In each of these examples, as with all epigenetic editing, the base DNA is left completely intact.
The clinic is the natural next step for epigenetic editing. Fortunately, many components of the epigenome editing workflow already exist in the clinical space, making the activation energy required for this transition relatively low. The delivery systems — such as viral vectors and lipid nanoparticles — and the DNA targeting system are already functioning in some clinical capacity. While challenges remain, based on the unique advantages and promising pre-clinical data, epigenetic editing should be ready to enter the clinic within the next few years.
What will the world look like with epigenetic editing?
Epigenetic editing is a precise and versatile modality with far-reaching clinical potential and the ability to fundamentally reshape our understanding and control of gene regulation. This platform opens the door to treating complex multigenic diseases, effective tissue engineering, and fine-tuning immune cell differentiation.
We are on the precipice of a new era in genetic medicine. Epigenetic editing combines the power of multiplexing with the precise control of expression needed to expand the reach of gene therapies, enhance cell therapies, and enable genetic medicine. It is an exciting time in the epigenetics field! The combined efforts of clinicians and researchers, utilising the power of epigenetic editing, have an invaluable new approach for realising the promise of genetic medicine for those patients that need it most.
Author Bio:
Dr Blythe Sather is the Vice President, Head of Research at Tune Therapeutics. She is an established T-cell biologist with extensive experience working with industry-leading teams to design novel therapies. She is currently focused on using genetic tuning to enhance and develop cell and gene therapy products for clinical impact.
References
- Brunet E, Jasin M. Induction of chromosomal translocations with CRISPR-Cas9 and other nucleases: Understanding the repair mechanisms that give rise to translocations. Advances in Experimental Medicine and Biology. 2018;:15–25.
- Fulcoli FG, Franzese M, Liu X, et al. Rebalancing gene haploinsufficiency in vivo by targeting chromatin. Nature Communications. 2016;7(1).
- Akbari B, Ghahri-Saremi N, Soltantoyeh T, et al. Epigenetic strategies to boost car T cell therapy. Molecular Therapy. 2021;29(9):2640–59.
- Thakore PI, Kwon JB, Nelson CE, et al. RNA-guided transcriptional silencing in vivo with S. Aureus CRISPR-Cas9 repressors. Nature Communications. 2018;9(1).
- Liu XS, Wu H, Krzisch M, et al. Rescue of fragile X syndrome neurons by DNA methylation editing of the FMR1 gene. Cell. 2018;172(5).
- Klann TS, Black JB, Chellappan M, et al. CRISPR–cas9 epigenome editing enables high-throughput screening for functional regulatory elements in the human genome. Nature Biotechnology. 2017;35(6):561–8.
Related topics
DNA, Drug Delivery, Drug Development, Drug Discovery, Gene Therapy, Genetic Analysis, Genome Editing, T cells, Targets
Related organisations
Tune Therapeutics
Related people
Dr Blythe Sather