Flow cytometry – accelerating neuroscience research
Posted: 18 September 2017 | Pushpanathan Muthuirulan | No comments yet
Flow cytometry continues to evolve at a fast pace and provides neuroscientists with the ability to perform many highly-specialised assays simultaneously. It meets the demands of cutting-edge research in neuroscience that has allowed researchers to isolate particular neural cells from heterogeneous population and catalogue its molecular or physical features…
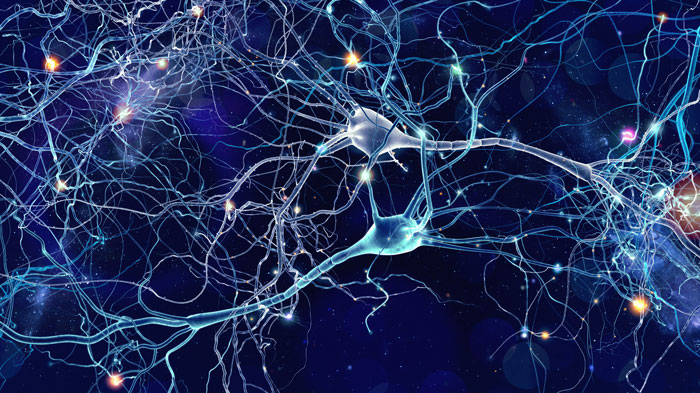
During the past decade or more, flow cytometry has proven to be one of the most powerful technologies used in research and clinics. It is a premier tool for isolation and enrichment of various cell populations based on their size, granularity and cell surface antigens.1 Flow cytometry has versatile applications in basic research, clinical practice and clinical trials. It has been routinely used in biomedical research, especially in the diagnosis of health disorders.2 Flow cytometers’ analytical capability to rapidly sort and purify a homogenous population of cells/particles of interests based on their optical properties have enabled this technology to hold a unique place among other biomedical technologies. Furthermore, the multiparametric capacity of flow cytometers to simultaneously and quickly collect multiple angles of scatter and different spectral components in large numbers of cells has enabled researchers to rapidly classify each cell into a cluster of similar cells. It is thus possible to discriminate cells/particles into clusters based onstatistical evaluation of the set of parameters collected for each cell/particle.3
Flow cytometry has tremendous applications in biological research that have impacted a wide range of fields from basic cell biology to neuroscience. Due to the overwhelming complexity and cellular heterogeneity of the nervous system, it is extremely difficult for researchers to obtain reliable molecular descriptions with cell-type specificity. Furthermore, the lack of adequate technologies for selective purification of the defined neuronal population from brain tissue has also hindered the comparative analysis of epigenetic regulation or molecular profiles of different neural cells.4 With rapid advancement in higher sensitivity instrumentation and greater throughput, flow cytometry has become a valuable tool for studying neural cells. In the recent past, it has been applied to a wide variety of heterogeneous tissues that has enabled researchers to understand cell type-specific attributes such as chromatin dynamics, nucleoproteins, protein-DNA/RNA interactions, and transcriptomes.5
Flow cytometry uses fluorescent probes to identify, quantify and even purify neural cells for prospective applications in neuroscience research. In general, cells can be identified based on surface antigen (receptor) expression or by intracellular expression of cytokines, chemokines and intracellular receptors.6 Flow cytometry rapidly measures fluorescence and other optical parameters of cells that have led to the aseptic isolation of viable cells at greater purity based on marker expression. Successful flow cytometric measurement relies on dissociation of neural tissue into single-cell suspension prior to fluorescent labelling and analysis. Magnetic-activated cell sorting (MACS) method is routinely used to enrich or purify cells of neuronal origin, which has greatly accelerated neuroscience research.7
Given its impressive capabilities, flow cytometry is crucially important in neuroscience research because it offers tremendous flexibility and the power to detect neural cell abnormalities. This article mainly covers the potential application of flow cytometry in neuroscience research, including isolation of neural cell types from a heterogeneous cell population, sorting of activated neurons, neurochemical/receptor profiling and assessment of intracellular changes in neurons, and determination of neural morphology and cell density changes during neurological conditions.
Isolation of neural cell types
Fluorescence-activated cell sorting (FACS) has been used to identify and purify selected neural cell types from brain tissues. This procedure involved dissociation of intact cell bodies from brain tissues, followed by sorting of dissociated neurons using commercially available antibodies against intracellular and extracellular proteins.8 This procedure has allowed high throughput and rapid quantification of molecular abnormalities in identified cell types with widespread applications in neuroscience research.
Alternatively, sorting of neural cell types can be carried out using transgenic animal expressing green fluorescent protein (GFP) in desired neurons controlled by a neuron-specific enhancer or promoter. However, this approach requires a generation of transgenic animals, which prohibits its applications across the whole animal kingdom.9 The use of flow cytometry in neuroscience has also been limited to embryonic and larval brain tissue, cultured cells, stem cells and synaptosomes.10 Thus, the separation of specific neural cell types from brain tissue based on intracellular markers permits potential applications of flow cytometry technology to many well-established disease models towards understanding brain disorders.
Sorting of activated neurons
C-fos is a proto-oncogene highly expressed within neurons upon depolarisation and has been widely used as a marker for neuronal activation. FACS can be used to analyse and purify fos-expressing neurons (activated neurons) from different areas of the brain. In the FACS procedure, brain tissue is dissociated into a single-cell suspension using mechanical or enzymatic methods. The dissociated cells were then labelled with fos antibodies and sorted according to their light scattering and immunofluorescent characteristics.11 In previous studies, FACS has been used to assess unique alterations of drug-induced gene expression in both activated and non-activated neurons.12 Thus, the c-fos activation of neurons can potentially demonstrate the causal roles of activated neurons in different behaviours. The major limitation associated with c-fos promoter-based techniques includes its ability to identify only sufficiently activated neurons, but not groups of neurons that are inhibited or insufficiently activated by cues for c-fos promoter activation. The absence of c-fos activation does not necessarily indicate that neurons are not active or have no role in behavioural phenotype.13 Thus, FACS sorting of activated neurons has been used to selectively target and study activated neuronal ensembles in behavioural models.
Neurochemicals and receptor profiling
Neurotransmitters are chemical messenger relaying signals from one nerve cell to another within the brain. These chemicals interact with target sites in nerve cells called neurotransmitter receptors located through the brain and mediate a wide range of behavioural processes.14 The imbalance between neurotransmitters and neurotransmitter receptors is an underlying cause of improper communication between nerve cells within the brain. Recently, scientists have been measuring the neurotransmitters and their receptors to correlate them with neurological conditions. Several fluorescent probes or antibodies are commercially available for detection and quantification of different neurotransmitters (eg, serotonin, dopamine, GABA, glutamate, histamine, nicotine) and neurotransmitter receptors (eg, NMDA receptors, nicotinic acetylcholine receptor, muscarinic acetylcholine receptors, GABA receptors and serotonin receptors) in nerve cells.
High-throughput flow cytometry live cell-based assay can be used to reliably detect the fluorescent antibodies directed against specific neurotransmitters or neurotransmitter receptors.15 The use of high-throughput flow cytometry cell-based assays is the most simple, quantitative, efficient and high-throughput approach to rapidly diagnose large patient cohorts in lesser time durations. Furthermore, the discovery of novel antibody biomarkers will enable more accurate diagnosis for accelerated treatment for neurological disorders.
Monitoring neuronal intracellular changes
Several fluorescent dyes are available to monitor intracellular changes such as calcium influx, ROS concentration, and viability of dissociated neurons using flow cytometry. The changes in intracellular Ca2+ concentration are among the most rapid responses associated with the excitability of nerve cells and they play a critical role in the generation of action potentials. The role of intracellular calcium response in nerve cells has also been associated with a wide range of intracellular signalling and regulatory pathways; however, the full implications of a change in intracellular calcium concentration are not well understood. With the use of a flow cytometer, measurement of dynamic Ca2+ concentration becomes accessible in neuronal populations using cell-permeable calcium-sensitive fluorescent dyes – thus providing valuable tools for studying nerve cell responsiveness.16,17 The oxidative stress that occurs with a generation of reactive oxygen species (ROS) is a major cause of neuronal cell death in neurodegenerative diseases. Elevated intracellular Ca2+ concentration is the most important trigger for ROS generation, the accumulation of which leads to cell death.18 Flow cytometry’s ability to utilise fluorescent probes such as 2,7-dichlorofluorescein diacetate (DCF-DA) and propidium iodide (PI) offers great promise to study the causal relationship between ROS generation and cell viability under various injurious stimuli.18,19 Thus, flow cytometry offers a broad platform from which to study intracellular changes in neurons towards understanding pathophysiology of neurological disorders.
Neural morphology and cell counting
Imaging flow cytometry offers a powerful tool to study neural cell morphology by a combination of visual analysis capabilities of microscopy and flow cytometry. In recent years, this technology has also been successfully used to identify differences in survival motor neuron expression during spinal muscular atrophy.20 The number of neurons and non-neurons across the brain can be counted using an isotropic fractionator method combined with flow cytometry (flow fractionator). For neural cell counting, a flow cytometer was used to estimate the proportion of NeuN+ nuclei to the total population of DAPI+ nuclei, which gives an exact quantification of neurons and non-neurons across the given brain region. The isotropic fractionator method combined with flow cytometry has been shown to rapidly produce accurate estimates of neuron number.21 Thus, flow cytometry is critically important in neuroscience research to study neuron morphology and neuron loss with normal ageing and by disease of the central nervous system.
Conclusion
To conclude, flow cytometry technology has provided critical impetus to modern science and is a cornerstone of current neuroscience research. Flow cytometry is a unique and valuable tool to study neural cells, forecasting the practical utility of this instrumentation on neuroscience research, with eventual emergence into the clinical diagnosis of neurological disorders. The ability of flow cytometry to physically sort different neuronal sub-populations from brain tissue further allows functional, morphological and molecular characterisation of pathogenesis mechanisms underlying neurological disorders. Thus, flow cytometry analysis should be implemented in prospective studies to optimise its applications as a tool to diagnose brain disorders.
Author Biography
Pushpanathan Muthuirulan is a postdoctoral researcher at the National Institutes of Health, working in the field of neuroscience, where his research focuses on developing tools to map complex neural circuits. He is a biologist with eight years of research experience in the discovery and development of drug candidates. Dr Muthuirulan has a BSc and MSc in Zoology from the American College and a PhD in Microbiology from Madurai Kamaraj University.
Address for correspondence
Laboratory of Gene Regulation and Development,
National Institute of Child Health and Human Development,
National Institutes of Health, Bethesda, MD 20892, USA
Email: [email protected]
References
- O’Donnell EA, Ernst DN, Hingorani, R. Multiparameter flow cytometry: advances in high resolution analysis. Immune network. 2013;13:43-
- Azari H, Sharififar S, Fortin JM, Reynolds BA. The neuroblast assay: an assay for the generation and enrichment of neuronal progenitor cells from differentiating neural stem cell progeny using flow cytometry.Journal of Visualized Experiments: JoVE. 2012;62.
- Adan A, Alizada G, Kiraz Y, Baran Y, Nalbant A. Flow cytometry: basic principles and applications. Critical Reviews in Biotechnology. 2017;37:163-
- Kuszynski C. Flow Cytometry in Neuroscience Research. InCurrent Laboratory Methods in Neuroscience Research. Springer New York. 2014;177-
- Okada S, Saiwai H, Kumamaru H, Kubota K, Harada A, Yamaguchi M, et al. Flow cytometric sorting of neuronal and glial nuclei from central nervous system tissue. Journal of Cellular Physiology. 2011;226:552-
- de Graaf MT, de Jongste AH, Kraan J, Boonstra JG, Smitt PA, Gratama JW. Flow cytometric characterization of cerebrospinal fluid cells.Cytometry Part B: Clinical Cytometry, 2011;80:271–
- Sassen WA, Lehne F, Russo G, Wargenau S, Dübel S, Köster RW. Embryonic zebrafish primary cell culture for transfection and live cellular and subcellular imaging. Developmental Biology. 2017; https://doi.org/10.1016/j.ydbio.2017.07.014
- Guez-Barber D, Fanous S, Harvey BK, Zhang Y, Lehrmann E, Becker KG, et al. FACS purification of immunolabeled cell types from adult rat brain.Journal of Neuroscience Methods. 2012;203:10–
- Lobo MK, Karsten SL, Gray M, Geschwind DH, Yang XW. FACS-array profiling of striatal projection neuron subtypes in juvenile and adult mouse brains.Nature Neuroscience. 2006;9:443.
- Arlotta P, Molyneaux BJ, Chen J, Inoue J, Kominami R, Macklis JD. Neuronal subtype-specific genes that control corticospinal motor neuron development in vivo.Neuron. 2005;45:207-
- Hitomi S, Kross K, Kurose M, Porreca F, Meng ID. Activation of dura-sensitive trigeminal neurons and increased c-Fos protein induced by morphine withdrawal in the rostral ventromedial medulla.Cephalalgia. 2017;37:407-
- Guez-Barber D, Fanous S, Golden SA, Schrama R, Koya E, Stern AL, et al. FACS identifies unique cocaine-induced gene regulation in selectively activated adult striatal neurons.Journal of Neuroscience. 2011;31:4251-
- Cruz FC, Koya E, Guez-Barber DH, Bossert JM, Lupica CR, Shaham Y, Hope BT. New technologies for examining the role of neuronal ensembles in drug addiction and fear.Nature Reviews Neuroscience. 2013;14:743-
- Spitzer NC. Neurotransmitter Switching in the Developing and Adult Brain.Annual Review of Neuroscience. 2017;25:1-
- Amatoury M, Merheb V, Langer J, Wang XM, Dale RC, Brilot F. High-throughput flow cytometry cell-based assay to detect antibodies to N-methyl-D-aspartate receptor or dopamine-2 receptor in human serum.Journal of visualized experiments: JoVE. 2013; 81:e50935.
- Tárnok A, Ulrich H. Characterization of pressure‐induced calcium response in neuronal cell lines.Cytometry Part A. 2001;43:175-
- Vines A, McBean GJ, Blanco‐Fernández A. A flow‐cytometric method for continuous measurement of intracellular Ca2+ concentration.Cytometry Part A. 2010;77:1091-1097.
- Boldyrev A, Song R, Dyatlov VA, Lawrence DA, Carpenter DO. Neuronal cell death and reactive oxygen species.Cellular and Molecular Neurobiology. 2000;20:433-
- Boldyrev A, Bulygina E, Leinsoo T, Petrushanko I, Tsubone S, Abe H. Protection of neuronal cells against reactive oxygen species by carnosine and related compounds.Comparative Biochemistry and Physiology Part B: Biochemistry and Molecular Biology. 2004;137:81-
- Chapp AD, Driscoll KM, Behnke J, Shan Z, Chen Q. Acetate, an Ethanol Metabolite Increases Neuroinflammation and Neuronal Death: Implications in Ethanol Neurodegeneration.The FASEB Journal. 2017;31(1 Supplement):1061-
- Arakawa R, Arakawa M, Kanek K, Otsuki N, Aoki R, Saito, K. Imaging Flow Cytometry Analysis to Identify Differences of Survival Motor Neuron Protein Expression in Patients with Spinal Muscular Atrophy.Pediatric Neurology. 2016;61:70-
- Collins CE, Young NA, Flaherty DK, Airey DC, Kaas JH. A rapid and reliable method of counting neurons and other cells in brain tissue: a comparison of flow cytometry and manual counting methods.Frontiers in Neuroanatomy. 2010;4.
Related topics
Flow Cytometry, Neurosciences, Research & Development
Related organisations
National Institute for Health Research (NIHR), National Institutes of Health (NIH)
Related people
Pushpanathan Muthuirulan