Evaluation of therapeutic antibody discovery technologies
Posted: 17 September 2019 | Dr Nancy Lopez-Anton (Antibody Discovery Group at Oxford Genetics) | No comments yet
Protein therapeutics are a major class of biopharmaceuticals and monoclonal antibodies (mAbs) rule this domain. In this article, Dr Nancy Lopez-Anton outlines the key technologies employed in this important therapeutic discovery area; how they have evolved and what obstacles must be navigated to ensure future success.
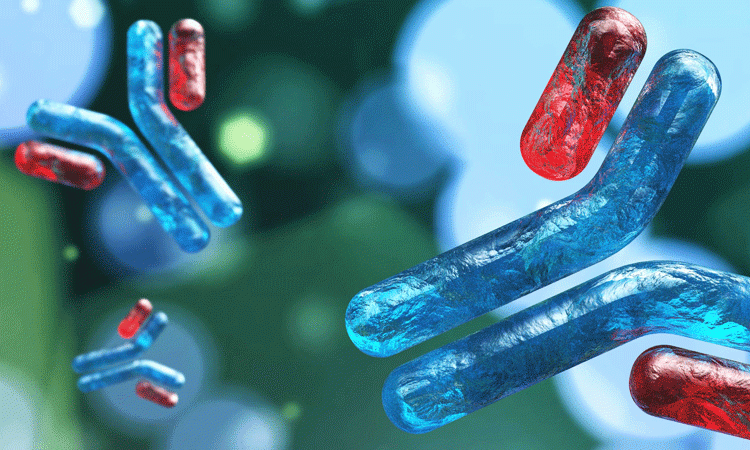
IN THIS ERA of promising therapeutic approaches such as genome editing and cell and gene therapy, mAb products are dominating the biopharmaceutical market.1 mAbs represent the most important class of protein therapeutics, delivering significant clinical benefit to millions of patients and yielding over $95 billion sales in 2017.2 At the end of 2018, over 80 mAb therapeutics had been granted marketing approvals and over 570 antibody therapeutics were at various clinical phases.3-5
Despite oncology being the primary application of therapeutic antibodies, immune-mediated disorders and infectious and neurological diseases have been therapeutically targeted by antibody agents. Indeed, adalimumab, the best-selling antibody drug of all time, is used for the treatment of rheumatoid arthritis. Approximately half of the late-stage pipeline of antibody therapeutics for cancer consists of immune checkpoint modulators or antibody-drug conjugates.
Desirable properties for therapeutic antibody products include high antigen-binding affinity, specificity, low immunogenicity, solubility, stability, manufacturability and adequate pharmacokinetics. Despite the huge developments in the antibody discovery arena over the last 40 years, some important limitations remain.
This article provides an overview of the main antibody discovery methods, focusing on the advantages, challenges and significant achievements of each technology.
Antibody discovery strategies
1. Hybridoma technology and transgenic animals
mAbs were initially generated by hybridoma technology, wherein animals are repeatedly immunised with the desired soluble target antigen. Spleen cells are then fused with myeloma cells to create immortal unique antibody-producing cell lines – hybridoma cells. This approach has garnered mAb reagents for research and diagnostics, enabling tracking, detection and quantitation of target molecules in cells and serum. The first approved therapeutic antibody, in 1986, was produced using this hybridoma technology.6 Muromonab-CD3 (Orthoclone OKT3®), which blocks CD3-mediated activation of T cells, was approved for use in preventing kidney transplant rejection.
However, the use of hybridomas for the generation of therapeutic antibodies soon demonstrated significant drawbacks, perhaps the most important of which was the immunogenicity of the murine antibody sequences. A significant percentage of patients who were administered this murine antibody developed anti-drug antibodies (ADA). In the late 1980s antibody engineering techniques were utilised to create chimeric and humanised antibodies to reduce this immunogenicity.3 First, murine constant domains were replaced by human sequences resulting in chimeric antibodies such as rituximab. Subsequently, the murine framework regions in the variable antibody domains were substituted with the closest human framework sequences, which resulted in the first humanised antibodies, including daclizumab and bevacizumab. Humanisation is still broadly used due to the accessibility and low cost of the hybridoma technology, which renders antibodies indistinguishable from fully human antibodies. In fact, the PD-1/PD-L1 targeting drugs pembrolizumab and atezolizumab are also humanised antibodies derived from mice.3
However, humanisation did not completely eliminate the possibility of an immune response to murine complementarity determining regions (CDRs).7 Transgenic mice or rats were therefore developed, in which murine heavy and kappa light chain genes were replaced with human germline sequences, thus producing fully human antibodies after immunisation.7,8 Many relevant therapeutic antibodies have been developed in transgenic animal platforms; denosumab, golimumab, ipilimumab and nivolumab, for example.
Despite their tremendous success, in vivo immunisation and hybridoma technology are not well suited for conserved, toxic and unstable antigens, especially transmembrane proteins.
2. Display technologies
In vitro selection technologies were developed in the early 1990s, which accelerated the discovery and engineering of fully human antibodies against almost every type of antigen with superior clinical efficacy and lower immunogenicity.9 Furthermore, as in vitro methods overcome immunological tolerance, antibodies or other affinity reagents that recognise highly conserved targets can be selected.
The basic principle of display technology is the direct physical link between the antibody gene (genotype) and the antibody product (phenotype). It allows the use of fully synthetic antibody libraries or libraries constructed from mRNA extract from natural sources, which can be either immunised or naïve.
Display technologies that generate binders in vitro offer the following unique advantages:
- the ability to carefully define selection conditions, allowing the derivation of antibodies recognising pre-defined epitopes or conformations
- the potential for high-throughput applications
- the immediate availability of genes encoding the selected antibody.
Together with the identification of new binders, display technologies enable the further improvement of selected antibodies.10,11
Together with the identification of new binders, display technologies enable the further improvement of selected antibodies”
Amongst these are phage, yeast and mammalian display; but, beyond the scope of this article, they also include bacterial display and acellular approaches (ribosome, mRNA, CIS-display and in vitro compartmentalisation). The success of display technologies has relied upon the conception and implementation of phage display, the expression of antibody fragments in bacteria, PCR-mediated amplification of antibody genes and libraries alongside large-scale next generation sequencing (NGS).12 NGS permits interrogation of display selection outputs to identify novel antigen-specific antibodies, giving a far greater coverage of sequence space than is permitted by random sampling based on standard colony picking and ELISA screens. Binders can then be screened for functional activity in biochemical and cellular assays.
2.1. Phage display
In 2018, George P Smith and Sir Gregory P Winter were jointly awarded the Nobel Prize in Chemistry for the development of phage display of peptides and antibodies.13
Phage display is the first and most widely used in vitro antibody selection technology with all the advantages of display technologies.9,14 It is an inexpensive, facile and powerful tool that allows isolation of recombinant mAbs in a short period of time. The subsequent modification of isolated antibody genes via mutagenesis can yield variants that are improved both in terms of specificity and affinity.11
In phage display (Figure 1), typically, single-chain variable domain (scFv) or fragment antigen binding (Fab) are expressed on filamentous phage through fusion with a viral coat protein.9 Each phage clone within the library displays unique antigen-binding sites on their surface. The antigen is immobilised onto plastic and the library is presented to it, before being biotinylated (covalent attachment of biotin to the antigen) in solution, followed by a ‘pull-down’ with streptavidin beads or expressed on the cell surface. Cell-based panning makes it possible to identify binders against unstable antigens such as membrane proteins, but is compounded by additional technical difficulties.15 The vast excess of non-binding antibody phage is removed by stringent washing and usually two to three panning rounds are required to isolate binders before screening for functional activity in biochemical and cellular assays.
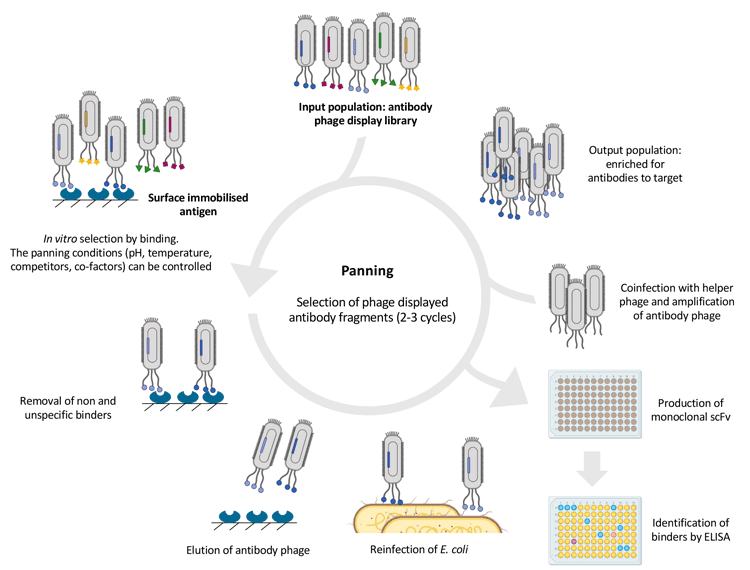
Figure 1: Overview of the phage display selection cycle. Antibody screening is usually carried out by ELISA. Adapted from references 9 and 11.
Blockbuster drugs such as adalimumab and ranibizumab have been developed using phage display. Adalimumab is the highest selling drug globally, with total sales of $411 billion since it was launched in 2002. Ranibizumab was obtained by affinity maturation of the Fab of bevacizumab using phage display.9 Moreover, approximately 35 percent of mAbs in clinical trials are derived from phage display.1,15
In phage display, positive clones are identified by bacterial colony picking and screening by ELISA, which does not distinguish between expression level and affinity.11 Therefore, a disadvantage is that the natural bacterial secretion machinery has limited capability in generating functional antibody fragments and antibodies generated by phage display often have poor biophysical properties compared to those produced in eukaryotes.
2.2. Yeast display
Yeast display was pioneered 20 years ago and has been used either alone or in conjunction with phage display. Similar to the phage, yeast display is suited for library screening and affinity maturation. Single chain Fv, Fab fragments, VHH-fragments of llama heavy-chain antibodies – known as nanobodies – or engineered Fc parts – called Fcab – can be effectively displayed on the yeast surface and screened.16,17
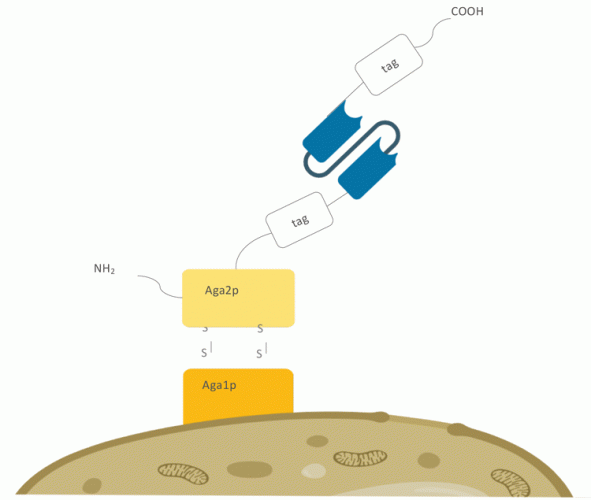
Figure 2: Yeast surface display setup on Saccharomyces cerevisiae. The antibody or protein
of interest is fused to the C-terminus of the Aga2p protein, which is covalently linked to the
core‑subunit Aga1p via two disulphide bonds.
Saccharomyces cerevisiae has been routinely used as a eukaryotic host for production of proteins that contain disulphide bonds, making use of the oxidative environment in the endoplasmic reticulum and the presence of chaperones18 (Figure 2). While yeast displayed libraries are smaller than phage libraries, the most significant advantage of yeast display is the quantitative and exhaustive survey of millions of cellular clones by fluorescence activated cell sorting (FACS). This enables the possibility to highly enrich binding clones and to select based on expression and affinity. However, the glycosylation, expression and secretion machinery of yeast is different from that of higher eukaryotes, which prompted the interest in creating a display system in higher eukaryotes.
2.3. Mammalian display
In mammalian display, antibodies are produced using the natural eukaryotic secretion machinery, enabling physiological post-translational modifications and folding, thereby improving their biophysical properties. Moreover, it is possible to display IgG directly on the cells in which the manufacture of antibodies is typically carried out, such as CHO cells. Several research groups and companies are developing mammalian display systems in antibody discovery, often used in combination with phage display acting as a quality control system. Mammalian display techniques have enabled the selection of antibodies with improved properties, including higher affinity,19 selectivity and manufacturability, as well as selection for function in cells expressing the antibody target.20 Compared to other strategies, however, a disadvantage of using cellular display is that only a relatively small library size (usually 107) can be interrogated. Additionally, as purified antigen must be applied to cells in solution or coupled to particles, the targets are restricted to stable, small and hydrophilic proteins.
Mammalian display techniques have enabled the selection of antibodies with improved properties”
Mammalian display approaches have evolved since its first description in 2006.21 The first step is the construction of the antibody library (full IgG, Fab or scFv) by cloning antibody genes from a suitable source, such as healthy donors, immunised patients or a secondary library from phage display. DNA was initially introduced into mammalian cells by standard transfection22,23 or electroporation methods. This approach risks introducing multiple antibody genes into each cell and poor efficiencies of delivery, thus reducing the proportion of cells containing the antibody library and resulting in the display of erroneous heavy and light chain combinations. Consequently, many passenger antibody genes may be isolated, thereby reducing the rate of enrichment of specific sequences. Solutions to this problem include the use of stable episomal vectors, the site-specific integration of transgenes using FLp recombinase23 (Flp-In system) and introduction of antibody genes by viral vectors.20 Recently, TALE nucleases or CRISPR-Cas9 have been used to direct the integration of the antibody genes into a single genomic locus.25
The cells displaying the antibodies (Figure 3) are presented to the target antigen in soluble format or bound to magnetic beads, creating an additional avidity of the antigens to allow selection of cells that express low affinity antibodies. The first mammalian display systems used adherent cells to express the antibodies,22,23 whereas later suspension cells,24,26,27 including CHO cells, were introduced.
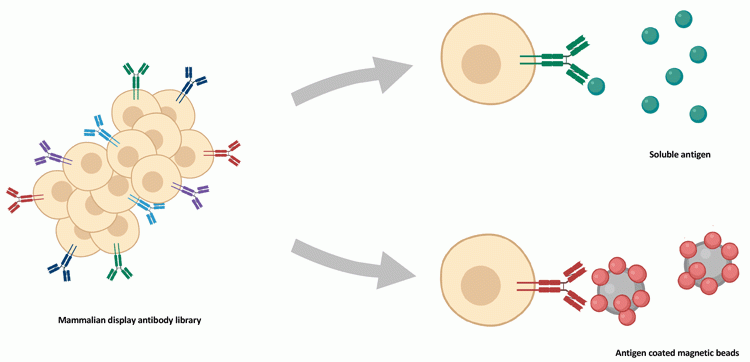
Figure 3: Screening strategy in mammalian display technologies. Antibody libraries expressed on mammalian cells are presented to soluble antigen (top) or antigen-coated magnetic beads (bottom). Antigen-binding cells are identified by flow cytometry using a directly- (ie, fluorescent) or indirectly‑labelled target.
It takes several rounds of fluorescence-activated cell sorting (FACS) to screen and select those cells expressing the antigen binders and candidate antibody sequences are identified by molecular biology techniques (PCR and NGS).
Conclusions and outlook
For over 30 years, antibody discovery technologies have provided the pharmaceutical market with therapeutic agents that have revolutionised the treatment of oncologic and autoimmune disorders. Importantly, display technologies have allowed the development of alternative scaffold-based affinity reagents that have emerged as a promising class of biomolecules with therapeutic potential.16 While engineered protein scaffolds can share some structural features of an antibody (eg, nanobody, scFv, single domains),4 scaffolds have also been described that are unrelated to mAbs (affibodies, anticalins, DARPins, alphabodies,28 dual-affinity retargeting molecules).
These proteins exhibit advantageous properties in comparison to full-length antibodies, such as improved tissue penetration, superior stability and cost-efficient production.29 Alternative scaffolds are often able to interact with cryptic or hidden epitopes that are difficult to address; some are even able to penetrate cell membranes and bind intracellular proteins, addressing proteins traditionally considered ‘undruggable targets’.
One of the remaining challenges facing the industry is the development of affordable therapeutics. Antibody production, manufacturability, formulation and shelf life that relate to the ‘developability’ of an antibody all have a dramatic impact on the cost of treatment. Improvements in these areas will importantly allow access of these therapeutics to a wider segment of the world’s population.
About the author
Dr Nancy Lopez-Anton is a Senior Scientist within the Antibody Discovery Group at Oxford Genetics. Nancy gained a Degree in Pharmacy and a Masters in the Management of Scientific Departments in the Pharmaceutical Industry at the University of Barcelona, Spain. She obtained her PhD in Pharmaceutical Biology at the Ludwig Maximilian University of Munich, Germany. Prior to joining Oxford Genetics in 2018 she worked on the development of magnetic beads-based diagnostic kits at Biokit and as project leader in Oryzon Genomics.
References:
- Ecker DM, Jones SD, Levine HL. The therapeutic monoclonal antibody market. mAbs 2015;7:9-14.
- https://www.marketwatch.com…
- Almagro JC, Daniels-Wells TR, Perez-Tapia SM, Penichet ML. Progress and challenges in the design and clinical development of antibodies for cancer therapy. Front. Immunol. 2018;8:1751. doi: 10.3389/fimmu.2017.01751.
- Elgundi Z, Reslan M, Cruz E, et al. The state-of-play and future of antibody therapeutics. Advanced Drug Delivery Reviews 2017;122:2-19.
- Kaplon H, Reichert JM. Antibodies to watch in 2019. mAbs 2019;11:219-238.
- Liu KHJ. The history of monoclonal antibody development – Progress, remaining challenges and future innovations. Annals of Medicine and Surgery 2014;3:113-116.
- Green L. Transgenic Mouse Strains as Platforms for the Successful Discovery and Development of Human Therapeutic Monoclonal Antibodies. Current Drug Discovery Technologies 2014;11:74-84.
- Brüggemann M, Osborn MJ, Ma B, et al. Human Antibody Production in Transgenic Animals. Arch. Immunol. Ther. Exp. 2015;63:101–108.
- Frenzel A, Schirrmann T, Hust M. Phage display-derived human antibodies in clinical development and therapy. mAbs 2016;8:1177–1194.
- Bradbury ARM, Sidhu S, Dübel S, McCafferty. Beyond natural antibodies: the power of in vitro display technologies. J. Nat Biotechnol. 2011;29:245–254.
- McCafferty J, Schofield D. Identification of optimal protein binders through the use of large genetically encoded display libraries. Current Opinion in Chemical Biology 2015;26:16–24.
- Parola C, Neumeier D, Reddy ST. Integrating high-throughput screening and sequencing for monoclonal antibody discovery and engineering. Immunology 2017;153:31–41.
- The Royal Swedish Academy of Sciences. Scientific background on the Nobel Prize in Chemistry 2018: directed evolution of enzymes and binding proteins. https://www.nobelprize.org/uploads/2018/10/advanced-chemistryprize-2018.pdf
- Frenzel A, Kügler J, Helmsing S, et al. Designing Human Antibodies by Phage Display. Transfus Med Hemother 2017;44:312–318.
- Alfaleh MA, Jones ML, Howard CB, Mahler SM. Strategies for selecting membrane protein-specific antibodies using phage display with cell-based panning. Antibodies 2017;6:10. doi:10.3390/antib6030010.
- Könning D, Kolmar H. Beyond antibody engineering: directed evolution of alternative binding scaffolds and enzymes using yeast surface display. Microb Cell Fact 2018;17:32.
- McMahon C, Baier AS, Pascolutti R, et al. Yeast surface display platform for rapid discovery of conformationally selective nanobodies. Nat Struct Mol Biol. 2018;25:289–296.
- Doerner A, Rhiel L, Zielonka S, Kolmar H. Therapeutic antibody engineering by high efficiency cell screening. FEBS Letters 2014;588:278–287.
- Bowers PM, Horlick RA, Kehry MR, et al. Mammalian cell display for the discovery and optimization of antibody therapeutics. Methods 2014;65:44–56.
- Zhang H, Wilson IA, Lerner RA. Selection of antibodies that regulate phenotype from intracellular combinatorial antibody libraries. PNAS 2012;109: 15728–15733.
- Ho M, Nagata S, Pastan I. Isolation of anti-CD22 Fv with high affinity by Fv display on human cells. PNAS 2006;103:9637-9642.
- Ho M, Pastan I. Mammalian Cell Display for Antibody Engineering. Methods Mol Biol 2009;525: 337–xiv.
- Zhou Y, Chen ZR, Li CZ, et al. A novel strategy for rapid construction of libraries of full-length antibodies highly expressed on mammalian cell surfaces. Acta Biochimica et Biophysica Sinica 2010;42:575-584.
- Zhou C, Jacobsen FW, Cai L, et al. Development of a novel mammalian cell surface antibody display platform. mAbs 2010;2:508-518.
- Parthiban K, Perera RL, Sattar M, et al. A comprehensive search of functional sequence space using large mammalian display libraries created by gene editing. MABS 2019 https://doi.org/10.1080/19420862.2019.1618673.
- Breous-Nystrom E, Schultze K, Meier M, et al. Retrocyte display technology: generation and screening of a high diversity cellular antibody library. Methods 2014;65:57–67.
- Beerli RR, Bauer M, Buser RB, et al. Isolation of human monoclonal antibodies by mammalian cell display. Proc Natl Acad Sci USA. 2008;105:14336-14341.
- Vaeck M. Alphabodies – working inside the cell. MedNous June 2014.
- Awwad S, Angkawinitwong U. Overview of Antibody Drug Delivery. Pharmaceutics 2018;10: 83. doi:10.3390/pharmaceutics10030083.
Related topics
Antibodies, Antibody Discovery, Biopharmaceuticals, Disease Research, Drug Development, Drug Discovery, Drug Targets, Monoclonal Antibody, Research & Development
Related conditions
rheumatoid arthritis
Related organisations
ForteBio, GenScript, Sartorius, Sphere Fluidics
Related people
George P Smith, Sir Gregory P Winter