Could GPCRs be causing people to respond differently to the same drug?
Posted: 16 October 2020 | Hannah Balfour (Drug Target Review) | 1 comment
Hannah Balfour explores how genetic variation in G-protein-coupled receptors (GPCRs) and the proteins that regulate the duration of G protein signalling could be contributing to disease and people’s divergent responses to the same therapeutics.
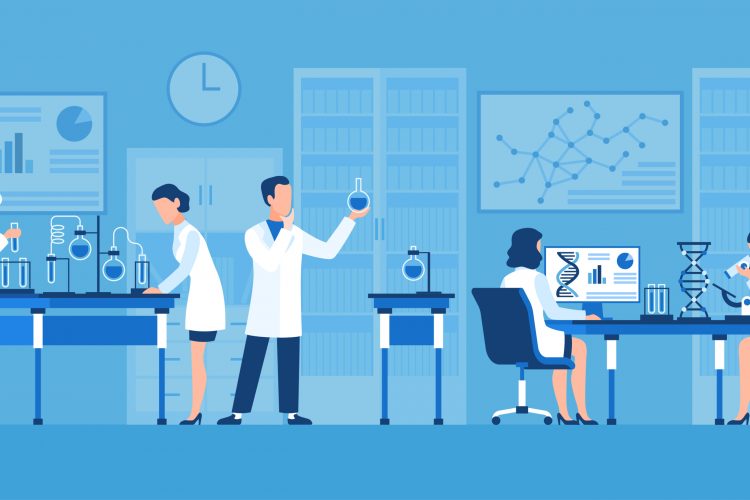
An introduction to GPCRs and RGS proteins
G-protein-coupled receptors (GPCRs) are one of the largest and most diverse families of proteins found in the mammalian genome.1 They primarily function to convert extracellular stimuli into intracellular signals, but because of their large number, widespread distribution and important roles in cell physiology and biochemistry, mutations in GPCRs are implicated in a range of acquired and inherited diseases.1
To date, over 600 inactivating and roughly 100 activating mutations in GPCRs have been linked with more than 30 human diseases,2 including endocrine disorders such as hypothyroidism, fertility disorders (hypogonadisms),3 cancers and neurodegenerative diseases like Alzheimer’s, to name just a few.
As of November 2017, an estimated 35 percent of drugs approved in the US or European Union (EU) targeted GPCRs.4 According to the same researchers, these drugs were directed at 134 different GPCRs, with GPCRs and GPCR-related proteins – ie, proteins upstream or downstream of GPCRs – representing roughly 17 percent of all protein targets for approved drugs (GPCRs alone account for 12 percent).
There is no consensus on the total number of GPCRs encoded for in the human genome and estimates vary widely, but genes for GPCRs could account for as much as one percent of the total human genome.5 Despite this, studies agree that their diversity is huge. According to Roth et al.,1 “the diversity of GPCRs is dictated both by the multiplicity of stimuli to which they respond, as well as by the variety of intracellular signalling pathways they activate.” External stimuli for these include environmental factors like light and smell, as well as chemical/molecular signals such as hormones, proteins, lipids and neurotransmitters.
GPCRs are cell surface proteins that couple to a G protein heterodimer inside the cell. G proteins consist of a Gα subunit and a βγ heterodimer. In the resting state, the Gα subunit binds guanosine diphosphate (GDP), forming Gα-GDP. When an agonist interacts with a GPCR, it induces a change in the conformation of the receptor that causes the exchange of GDP for guanosine triphosphate (GTP). As a result, the Gα subunit of the G protein uncouples from the βγ heterodimer and both go on to trigger various downstream signalling events, which depend on the makeup of the G protein coupled to the receptor. There are 18 known Gα subunits, five different β and 11 γ variants.1 To stop signalling, GPCRs can be internalised, facilitated by arrestin binding.
![GPCR signalling [Image modified from "Signaling molecules and cell receptors: Figure 5," by OpenStax College, Biology (CC BY 3.0).]](https://www.drugtargetreview.com/wp-content/uploads/GPCR-signalling-diagram-584x500.png)
[Image modified from “Signaling molecules and cell receptors: Figure 5,” by OpenStax College, Biology].
RGS proteins
GPCR signalling can also be terminated by regulator of G protein signalling (RGS) proteins, which are an emerging family of GTPase-activating proteins (GAP) that can accelerate the hydrolysis of GTP bound to the Gα subunit by two orders of magnitude. Hydrolysis of GTP converts it back to GDP, inactivating the Gα subunit and causing re-association with the βγ heterodimer, thus terminating the downstream signalling effects of both Gα-GTP and βγ.6
The RGS protein shutoff mechanism limits G-protein signalling to a brief time window, allowing cells to reset and accept new incoming signals. Without it, the GPCR-initiated signal remains active and signalling becomes dysfunctional.
RGS proteins as drug targets
A growing body of literature has described the roles of RGS proteins in a variety of conditions, including cancer, inflammation, cardiovascular processes and neuroinflammation. According to O’Brien et al.,7 targeting RGS proteins, instead of the upstream GPCRs, has advantages for drug specificity because different tissues expressing non-unique GPCRs (ie, those expressed in many cells/tissues) employ specific RGS proteins to control the signalling processes. Therefore, targeting the unique regulatory proteins would provide an increased level of specificity over targeting the GPCRs directly.
In a new paper,8 Scripps Research Institute scientists sought to understand the principles that govern the selectivity of Gα regulation by RGS proteins. The team mapped how RGS proteins regulate GPCR-initiated signals and found that people may respond differently to the same drugs because they express different variants of RGS proteins. As a result of the divergent proteins, their cells may respond differently to stimuli activating the same GPCRs.
…the natural variation to RGS proteins observed within the population may be causing people to react differently to the same GPCR-targeting drugs”
The study’s senior author Dr Kirill Martemyanov, professor and chair of the Department of Neuroscience at Scripps Research’s Florida campus, said that their study established how RGS proteins function normally and how they are implicated in disease to provide a basis for fixing the disorders they are associated with.
The researchers suggest that RGS proteins serve as a ‘reset button’ for cellular receptors and that without this function, cells are unable to stop passing along old signals and simultaneously prevented from picking up new ones. Martemyanov explained this phenomenon in the context of RGS regulation loss in the light-detecting cells of the retina: “Patients born with this condition cannot stop perceiving light, even when they go into a dark room and they cannot track moving objects very well because they lack the normal visual refresh rate.” He added that this loss of RGS protein regulation could be particularly devastating if it occurs in either the heart or the brain, where timing is vital for function.
Methods and findings
In their study, Martemyanov and colleagues studied how each of the 20 canonical RGS proteins found in human cells selectively recognises and regulates its G protein counterparts using real-time kinetic measurements in living cells. The data reveals the rules underlying RGS-Gα subunit recognition and creates a map of how GPCR signals are routed in cells.
The study’s first author Dr Ikuo Masuho, staff scientist in the Martemyanov lab, explained: “This selective recognition of G protein subunits turns out to be performed by a few elements in each RGS protein – elements organised in a pattern resembling a barcode.”
Through their analysis of more than 100,000 genomes, the team identified mutations and common variations in RGS barcode regions which were able to disrupt Gα subunit recognition by RGS proteins. These mutations could either prevent recognition of Gα subunits, eg, a mutation in RGS16 which has been linked to insomnia or cause RGS proteins to incorrectly recognise the wrong subunit.
![Diagram showing how RGS barcode variability changes the selectivity of the proteins [Credit: Martemyanov et al.].](https://www.drugtargetreview.com/wp-content/uploads/RGS-Protein-selectivity-genetic-diversity-diagram.jpg)
Diagram showing how RGS barcode variability changes the selectivity of the proteins [Credit: Martemyanov et al.].
Martemyanov said: “It is clear that genetic variation in the RGS barcode regions has the potential to disrupt normal GPCR signalling, to cause disease or to create more subtle differences or traits… it may help explain why different individuals treated with the same GPCR-targeting drug often differ widely in their responses.”
According to the authors, the RGS barcode regions – and the Gα subunits they regulate – are continuously evolving. The researchers compared the analyses of different species of RGS proteins to create less nuanced, “ancestral” RGS proteins, which enabled them to devise principles for developing novel recombinant RGS proteins that could be used to regulate a desired set of G proteins.
These same principles could be used to guide the development of drugs targeting RGS proteins and treatments that put corrective new RGS proteins in cells might be another avenue for potential therapies, said Martemyanov.
Further research
Martemyanov’s lab is interested in understanding the dynamics of G protein signalling regulation in two neuronal systems:
- the basal ganglia, where GPCRs co-ordinate movement and may be implicated in disorders like Parkinson’s, Huntington’s and Tourette Syndrome. They may also mediate reward behaviour, potentially contributing to the addictive effects of opioids and cocaine.
- the retina, where G protein-mediated signalling co-ordinates inputs from photoreceptors and sensory neurons to enable sight. Macular degeneration and congenital night blindness are two examples of retinal dysfunction that may be caused by GPCRs and GPCR-associated proteins.
Conclusion
The diversity and ubiquitous nature of GPCRs and their RGS proteins mean that they are implicated in a wide range of pathologies, from neurodegeneration to sexual dysfunction and cancer. However, despite their promise, GPCRs are not necessarily the most attractive drug targets as many are expressed throughout the body, limiting any potential drug’s specificity and increasing the likelihood of off-target effects.
As a result, GPCR-associated proteins may be more fruitful – especially in the case of the RGS proteins, where individual cells employ unique regulators for non-unique GPCRs.
In their study, Martemyanov and colleagues characterised how 20 human RGS proteins recognise and regulate their respective Gα subunits. They established that RGS proteins recognise Gα through a region that has a barcode and that some of the genetic mutations identified in this region were able to disrupt or drive incorrect Gα subunit recognition. Additionally, the natural variation to RGS proteins observed within the population may be causing people to react differently to the same GPCR-targeting drugs, which is potentially a further argument for targeting specific RGS proteins, rather than their upstream receptor.
References
- Kroeze, W., Sheffler, D. and Roth, B. G-protein-coupled receptors at a glance [Internet]. Journal of Cell Science. 2003. [Cited: 6 October 2020] Available at: https://jcs.biologists.org/content/116/24/4867
- Schöneberg, T., Schulz, A., Biebermann, H. et al. Mutant G-protein-coupled receptors as a cause of human diseases [Internet]. Pharmacology & Therapeutics. 2004. [Cited: 6 October 2020] Available at: https://pubmed.ncbi.nlm.nih.gov/15556674/….
- GPCRs Diseases [Internet]. Creative BIOMART. [Cited: 6 October 2020] Available at: https://www.creativebiomart.net/gene-family/8-gpcrs-diseases.htm
- Sriram, K. and Insel, P. GPCRs as targets for approved drugs: How many targets and how many drugs? [Internet]. Molecular Pharmacology. January 2018. [Cited: 6 October 2020] Available at: https://molpharm.aspetjournals.org/content/early/2018/01/03/mol.117.111062
- Cook, J. G Protein–Coupled Receptors as Disease Targets: Emerging Paradigms [Internet]. The Ochsner Journal. 2010. [Cited: 6 October 2020] Available at: https://www.ncbi.nlm.nih.gov/pmc/articles/PMC3096185/
- Roman, D. and Traynor, J. Regulators of G protein signaling (RGS) Proteins as Drug Targets: Modulating GPCR Signal Transduction [Internet]. Journal of Medicinal Chemistry. September 2011. [Cited: 6 October 2020] Available at: https://www.ncbi.nlm.nih.gov/pmc/articles/PMC3208131/
- O’Brien, J., Wilkinson, J. and Roman, D. Regulator of G-protein signaling (RGS) proteins as drug targets: Progress and future potentials [Internet]. Journal of Biological Chemistry. October 2019. [Cited: 7 October 2020] Available at: https://www.ncbi.nlm.nih.gov/pmc/articles/PMC6901330/
- Masuho, I., Balaji, S., Muntean, B. et al. A Global Map of G Protein Signaling Regulation by RGS Proteins [Internet]. Cell. October 2020. [Cited: 7 October 2020] Available at: https://www.cell.com/cell/fulltext/S0092-8674(20)31140-5?…
Related topics
Disease Research, Drug Targets, Genomics, GPCRs, Protein, Proteomics, Sequencing
Related conditions
Alzheimer's disease (AD), Cancer, Hypothyroidism, Neurodegenerative diseases
Related organisations
The Scripps Research Institute (TSRI)
Related people
Dr Ikuo Masuho, Dr Kirill Martemyanov
I do believe all of the concepts you have introduced for your
post. They are really convincing and can definitely work.
Nonetheless, the posts are very short for starters. May you please prolong them a little
from next time? Thank you for the post.