3D bioprinting COVID-19 lung models for drug development
Posted: 19 June 2020 | Hannah Balfour (Drug Target Review) | No comments yet
Keith Murphy, CEO of Viscient Biosciences, discusses the process of developing a 3D bioprinted tissue model and their potential in developing therapeutics for COVID-19 and other diseases.
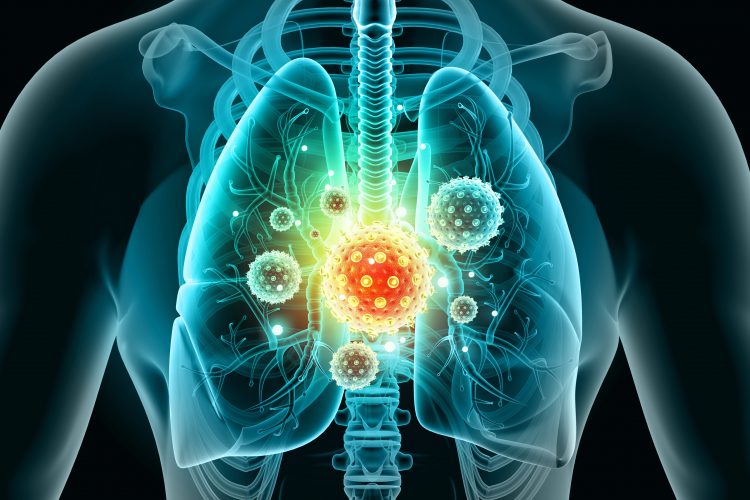
Drug Target Review’s Hannah Balfour questioned Keith Murphy, Chief Executive Officer of Viscient Biosciences LLC, about how the company goes about developing three-dimensionally (3D) printed organ models and their work to model COVID-19 infection in the lung to aid in drug development.
What are the advantages of using 3D tissue models over traditional 2D cell and animal models of disease?
Firstly, 2D models work very well for studying certain cells types; skin cells, for example, survive well in a flat 2D surface because it closely mirrors how they are in the body. We have discovered many therapeutic options for diseases in these cell types using these models. But many other cell types do not thrive in this context. Liver cells, for instance, stop functioning within 48 hours when they are cultured in a petri dish, so it is almost impossible to study their true biology this way.
Animal models are similarly useful in many contexts; some models closely recapitulate human disease, and for these we have been very productive in finding therapeutics. However, the genetic differences between animals and humans continue to cause problems – again, the liver is an example of where animal models are insufficient.
Our thinking is that 3D tissues are the intersection of the other two, both because they can be made as a 3D tissue comprised of fully human cells and also due to the possibility of taking these cells directly from patients, so that the model closely represents human disease.
What 3D tissue models are Viscient currently developing?
We are working on building a lung tissue model that can be used in two ways:
- For infectivity research, to aid our understanding of how the COVD-19 coronavirus infects cells in the airway; and
- Screening potential therapies – the more closely our model recreates the human disease, the better our understanding of whether a therapy will work.
There are numerous pharmaceutical companies currently developing therapies and our goal is to work together to help identify potential lead drug candidates.
How do you design a 3D tissue model for bioprinting?
When developing a 3D model, you first need to study the native tissue you are aiming to recreate. You have to understand what cells are present, how they are positioned relative to one another and what ratios they are present in.
Finding the correct cell types is often a huge challenge and one way of achieving it is to take cells from patients with the disease, as we have shown with our non-alcoholic steatohepatitis (NASH) liver model. These are the best possible representations of the disease and its genetic underpinnings. Ideally, we would like to do this with our COVID-19 lung model, but due to the highly infective nature of the tissue there are limitations and controls required for us to handle those appropriately.
Another consideration is ‘what conditions are required for these cells?’ Unlike 2D cell models, 3D tissue models typically contain multiple cell types, for example, the NASH liver model uses four different cell types. Interestingly, we have found that when we worked with multiple cell types we did not need to provide as many growth factors, because the cells support each other as they would in the body, supplying many of the required growth factors. There is natural crosstalk occurring that, if you take one cell type in isolation, you need to replace.
What indicators are there that a tissue model accurately recapitulates the disease?
There are two key methods for assessing how closely the model reflects the clinical sample of a patient. One is looking under the microscope at the histology: in the case of the NASH liver model, the disease is traditionally diagnosed by biopsy of the liver in which we look for collagen fibrosis in the tissue, as well as small, spherical droplets of lipid that build up as fat deposits. Our 3D bioprinted model also has these pathologies when examined under the microscope. Importantly, NASH collagen fibres form in a specific pattern, which differentiates it from other related diseases, and it is matched by the pattern seen in the model.
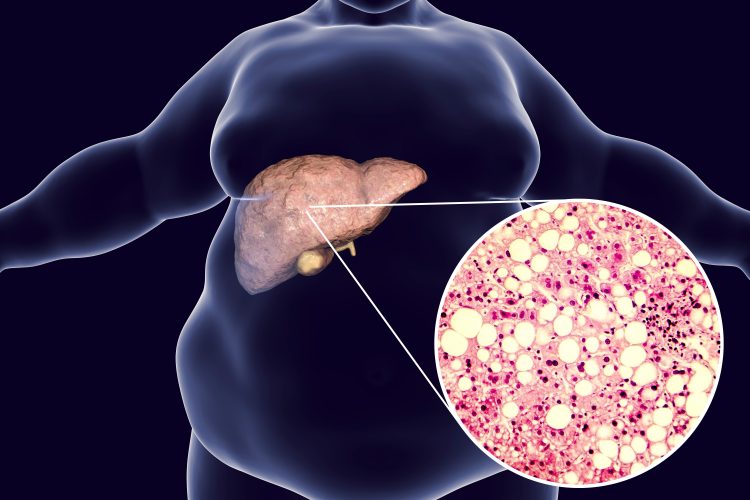
3D illustration and photomicrograph of liver steatosis.
The second is to explore which genes are expressed and whether the expression patterns match. The gene expression pattern in our bioprinted NASH model compares much more favourably than those of the animal models that are out there. For both these reasons we believe it provides a good picture of NASH.
How can a 3D bioprinted model be used to identify potential drug targets?
We look at gene expression patterns and compare them to that of normal cells in that tissue type. We try to suggest which differences are driving the disease, as opposed to those that have variable expression as a result of the disease.
Once these have been identified using algorithms and experimentation, we can knock out those genes we believe are causing the disease. Take the case of NASH again, if we can knock out a gene and it dramatically reduces the amount of collagen fibres that develop in the tissue, then that gene is a target for a drug therapy.
What are you excited about in the field of tissue models?
the more closely our model recreates the human disease, the better our understanding of whether a therapy will work”
I am excited about the potential for human cell models to replace animal models, and beyond that, how they can contribute to personalised medicine. Each of these models (if they are built using patient tissue samples) is essentially a mini clinical trial in which each patient can be studied separately. Theoretically, you could take cells from hundreds of donors, make bioprinted tissues for each and run the drug that you want to test to get a clear idea of how it works in a population before investing in a costly clinical trial.
For example, when performing the bioprinted tissue testing, you may find a drug targeting a particular gene or protein works well in 60 percent of your sample population, but in the other 40 percent it has no effect. This information would allow pharma to design a clinical trial in which there is a greater chance of success, rather than a trial where 40 percent of the patients did not respond, for instance by requiring that participants have a specific gene expressed that makes them likely to benefit from treatment.
The other advantage of this approach for pharma is they can start the process from the beginning in the 40 percent who did not respond and identify what is different in their particular disease, and hopefully find a drug to combat it.
Essentially, 3D tissue models could cut costs and time taken to develop new drugs by enabling marker-based therapy selection and reducing the rate of failure in clinical settings.
Related topics
3D printing, Bioprinting, Cell-based assays, Disease Research, Drug Development, Drug Targets, Genomics, In Vitro, Organoids, Research & Development
Related conditions
Coronavirus, Covid-19, Non-alcoholic steatohepatitis (NASH)
Related organisations
Viscient Biosciences LLC
Related people
Keith Murphy (Viscient Biosciences)