The promise of iPSC-based multi-cell therapeutics
Posted: 5 July 2023 | Taylor Mixides (Drug Target Review) | No comments yet
Drug Target Review’s Taylor Mixides exclusively interviews Matt Angel, PhD, Chief Executive Officer and President of Eterna Therapeutics, about a potential new class of cell therapies that may play an important role in the development of new cancer treatments.
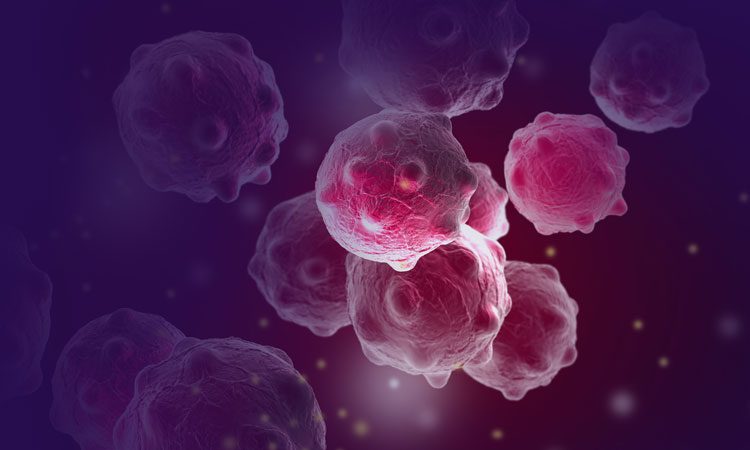
Induced pluripotent stem cell (iPSC) therapies have the potential to treat a wide variety of devastating diseases. iPSC-derived lymphocytes, eg, T cells and natural killer (NK) cells, engineered to express targeting molecules such as chimeric antigen receptors (CARs) have shown clinical promise to treat haematological malignancies. More recently, iPSC-derived myeloid cells are being developed to treat both haematological malignancies and solid tumours due to their ability to infiltrate and modulate the tumour microenvironment. While iPSC therapies hold great promise, several challenges remain, including poor infiltration of cytotoxic lymphocytes into solid tumours and insufficient cytotoxicity of myeloid cells.
Please summarise Eterna Therapeutics’ ($ERNA) new research at ASGCT on a cell therapy approach that integrates multiple cell types to emulate the natural immune system’s fight against diseases?
Our new research outlines an approach that aims to treat cancer, including solid tumours, with a mixed-cell-type cell therapy, comprising both myeloid and lymphoid cells derived from engineered iPSCs. The data demonstrate an approach of taking a pluripotent stem cell (PSC) and engineering it to generate a therapeutic comprising two different types of cells differentiated from those iPSCs that have been engineered to work synergistically. It’s comprised of myeloid cells that are inherently adept at infiltrating solid tumours and lymphoid cells that are very good at killing cancer cells directly.
With this data we’re showing what we believe to be the first approach involving mixing these two cell types in a single cell therapy to treat solid tumours.
Could you provide more details about this therapeutic: how does it emulate the natural immune system’s response to diseases?
The natural immune system fights precancerous lesions and prevents the body from developing cancer through the concerted effort of multiple cell types acting in synergy. In the case of solid tumours, myeloid cells called macrophages are adept at infiltrating solid tumours, while lymphoid cells, such as T cells and natural killer (NK) cells are efficient at engaging with cancer cells and killing them directly.
solid tumours, myeloid cells called macrophages are adept at infiltrating solid tumours
However, in many cases, the natural immune system is unable to effectively address solid tumours because the microenvironment of a tumour is incredibly hostile to immune cells beyond a certain stage. Our approach takes iPSCs that are engineered in such a way that when they are differentiated into cells that replicate a portion of the natural immune system, including both myeloid and lymphoid cells, we can amplify each cell types’ effectiveness. For example, we are able to enhance the capability of macrophages to recruit lymphoid cells by engineering them to secrete cytokine gradients that directly recruit lymphoid cells to the site of the solid tumour. Thus, our strategy aims to emulate the natural immune system in the way that it fights cancer and also enhances key functional features of those cells, so that the therapeutic is even more effective than the natural immune system.
In the study presented at ASGCT, what were the specific findings regarding the synergistic killing of SKOV3 ovarian tumour cells by the iPSC-derived lymphoid and myeloid cells? Did the ROR1-targeted myeloid cells show any specific advantages or improvements in targeting and engaging with solid tumours?
The data presented at ASGCT showed that a mixed-cell-type therapy comprising both macrophages and NK cells can be more effective at killing SKOV3 ovarian tumour cells than either macrophages or NK cells alone. The level of cell killing was greater than twice the amount of killing observed with either cell type alone. This suggests that these cells acted synergistically to create this enhanced ovarian tumour cell-killing phenomenon that we observed and that we presented in this study.
mixed-cell-type therapy comprising both macrophages and NK cells can be more effective at killing SKOV3 ovarian tumour cells
Related to the ROR1-targeted myeloid cells, we showed engineering of macrophages by administering mRNA encoding an ROR1-targeting CAR. Our data indicate that for cells expressing the CAR, the CAR was functional and successfully bound to ROR1 target protein. We believe this lays the groundwork for engineering these kinds of myeloid cells to potentially target any cancer antigen.
What are the key advancements in nucleic-acid delivery and targeted gene insertion that Eterna and Factor Bioscience presented at the ASGCT? How do these advancements contribute to the development of mRNA cell engineering therapies?
Eterna and Factor Bioscience presented several advancements in nucleic acid delivery, including a new family of mRNA formulations incorporating ionisable cationic lipids that can deliver nucleic acids into cell types that are traditionally understood to be refractory to lipid-based delivery, in particular to blood cells.
We’re very excited about this result, as it may be useful for the development of in vivo mRNA cell engineering approaches to change a cell’s phenotype by delivering nucleic acids to cells to engineer blood cells in the body directly. Just as the field has seen success with autologous CAR T-cell therapies for cancer, these kinds of novel blood cell-targeting delivery systems give us the tools to potentially develop approaches to do the same thing that CAR-T therapies do, except directly within the patient’s body.
In terms of targeted gene insertion, we presented data on a new gene-editing endonuclease called UltraSlice™ nickase, which is a novel protein-based gene editing system that is very different to CRISPR, as it does not involve a guide RNA. Here, the specificity of the protein is built into the gene-editing protein itself and, unlike earlier generation gene-editing systems, this system is not designed to create double-strand breaks in genomic DNA, but rather is designed to create nicks or single-strand breaks in genomic DNA.
One of the results that we presented, which was very surprising, is the high efficiency at which these nickases can promote insertion of desired sequences into defined genomic DNA loci without creating double-strand breaks. This is of enormous interest when it comes to inducing engineering cells to overexpress protein, or a combination of proteins, in a controlled manner. In those applications there is a need to insert a DNA sequence encoding a protein of interest in a highly controlled, very precise way, into a defined region or target site in the genome of a living cell without creating off-target effects. Those are unwanted mutations elsewhere in the genome of the cell that could potentially cause the cell to become cancerous. Those kinds of mutations are often associated with double-strand breaks in DNA. With nickase functionality designed to create single strand breaks, we can achieve targeted insertion without the risks of off-target, potentially cancer-causing, mutations that are associated with earlier generation gene-editing technologies.
The study aims to efficiently transfect blood cells. Can you elaborate on the potential applications of this platform for ex vivo and in vivo mRNA cell engineering, such as cell reprogramming, gene editing and protein replacement?
The ability to efficiently transfect blood cells is of enormous interest, as blood cells contain many of the immune cells – including T cells, NK cells and myeloid cells – that are being actively explored as the foundation of cell therapies to treat cancer. In addition to cancer, there are also applications in genetic disease, including heritable blood diseases such as sickle cell disease and beta thalassemia, where we can transfect a patient’s blood cells with mRNA-encoding gene-editing proteins to correct the disease-causing mutation. The correction is permanent in the treated cells, so we would anticipate that this approach could yield a durable effect in patients.
In cancer specifically, there has been a large push toward developing allogeneic CAR T-cell therapies that can be used ‘off-the-shelf’ with several candidates now in late-stage clinical trials. However, many allogeneic cell therapy candidates use viruses to transfect blood cells in order to introduce the CAR, but this viral transduction can be inconsistent and unreliable. Viral manufacturing is not trivial – it can be difficult to manufacture enough virus with consistent properties. Furthermore, any viral approach carries some risk of genomic disruption, which could cause cells to mutate and become cancerous.
With tools that allow us to effectively transfect blood cells, we now have completely non-viral approaches of introducing genes of interest into blood cells. This approach offers the potential of replacing current viral approaches with non-viral approaches involving lipid transfection of mRNA, either mRNA directly encoding a protein of interest for a transient expression of that protein or mRNA encoding gene-editing proteins for insertion of a gene of interest into the genomic DNA of blood cells for a more durable or longer-lasting expression of a transgene.
In cancer specifically, there has been a large push toward developing allogeneic CAR T-cell therapies that can be used ‘off-the-shelf’ with several candidates now in late-stage clinical trials.
In the field of cell reprogramming, there is potential for the new lipids that we’ve presented at ASGCT to facilitate the development of novel reprogramming processes using blood cells as the starting cell type. This may be advantageous as blood cells are readily accessible through non-invasive means and may also be useful in the case of reprogramming cells from patients with heritable or genetic diseases.
How does this methodology support the development of engineered cell therapies that express therapeutic proteins?
Engineered cell therapies that express therapeutic proteins fall into two categories – cell therapies that express therapeutic proteins transiently for days or weeks and those that express therapeutic proteins for weeks or months, or in the case of cell therapies designed to engraft in the patient for as long as the rest of the patient’s life. This methodology is useful in both categories of applications, allowing us to deliver mRNA encoding therapeutic proteins directly to blood cells, both ex vivo, outside the body, and in vivo, inside the body. In this way, we can directly express therapeutic proteins by transfecting blood cells with mRNA using the novel lipid delivery systems that we presented at ASGCT.
This is very exciting because the approach is completely non-viral and also DNA free, so we avoid using adeno-associated viruses (AAVs), lentiviruses, episomes or plasmids. Consequently, there is fundamentally no risk of genomic integration of the vector, since in this case the vector is mRNA and mRNA is translated into the therapeutic protein in the cytoplasm of cells. This approach has the potential to create a new class of therapies based on the delivery of mRNA directly to blood cells to express therapeutic proteins. In addition, this methodology also supports the development of gene-edited engineered cell therapies by allowing us to deliver, direct to blood cells, mRNA not encoding a therapeutic protein but encoding gene-editing proteins that enable us to insert a gene encoding the therapeutic protein into the DNA of the blood cell. In that way, we induce the blood cell to durably express the therapeutic protein for as long as the blood cell persists in the patient. This can be useful, for example, in the generation of engineered cell therapies that express cancer-targeting proteins such as CARs. In particular, CAR T approaches where the cells may proliferate inside the patient after they have been administered. With this approach there can be confidence that the cells, even as they’re dividing, will express the therapeutic protein. This differs greatly to a transient approach, where the vector would be diluted over time and the expression of the therapeutic protein would be expected to be reduced over time.
Regarding UltraSlice™ gene-editing proteins for targeted insertion of donor sequences; please explain how these gene-editing proteins improve the safety and precision of in vivo gene insertion compared to traditional gene-editing methods?
Traditional gene editing methods involve inducing a cell to express an endonuclease protein that creates double strand breaks in the genomic DNA of the cell. These breaks either result in a knockout mutation at a target site to inactivate a gene, or can be used to insert a new gene into the site of the double strand break at the target site. One of the challenges from a safety perspective of traditional gene-editing methods is their level of imprecision, known as ‘off-target effects’. While traditional gene-editing methods may be effective at creating a double strand break at a target site, they often also create double strand breaks elsewhere in the genome and it can be difficult to predict where those breaks may occur.
In the case of ex vivo therapies, off-target effects can be tolerated because the cells may be characterised sufficiently ex vivo to ensure that no important genes, like tumour suppressor genes, have been disrupted and that the cells have not been harmed in a way that might cause them to become cancerous before they’re reintroduced or introduced into the patient. However, those kinds of characterisation approaches are generally not available in the case of in vivo gene insertion, where gene editing takes place inside the patient’s body. In that case, if there are off-target effects in even one cell, that could generate mutations that cause that cell to become cancerous. There has been an extraordinary need to eliminate the potential of gene-editing tools to create these types of unwanted, possibly cancer-causing, mutations.
At Eterna, we are using a novel gene-editing protein that we call UltraSlice™ nickase, which is designed to create single-strand breaks where only one of the two strands of genomic DNA at the target site is cleaved, leaving the complementary strand completely intact. We have shown that when we co-deliver a donor DNA sequence for insertion into that target site it can be inserted at very high efficiency into the site of the single-strand break. In those cells where insertion does not occur, the single-strand break is repaired scarlessly. The nick is repaired by the cell leaving no trace of any disruption or mutation at the target site. This is in stark contrast to traditional gene-editing methods where if no insertion takes place, a double-strand break often leads to a deletion type mutation as several bases of genomic DNA are lost while the cell tries to repair the break. We are very excited about this advance and are looking forward to developing therapies using UltraSlice™ nickase across many areas of medicine.
Author Bio:
Matt Angel, PhD
Matt is currently the Chief Executive Officer and President of Eterna Therapeutics, where he leads Eterna’s commitment to realizing the potential of mRNA cell engineering to provide patients with transformational new medicines. In addition to his role at Eterna, Matt serves as Co-Founder, Chairman and CEO of Factor Bioscience Inc.
Related topics
Cell Regeneration, Cell Therapy, Cell-based assays, DNA, Drug Development, Drug Discovery, Drug Discovery Processes, Drug Targets, Genome Editing, In Vitro, Organoids, T cells, Targets
Related conditions
Cancer
Related organisations
eterna therapeutics
Related people
Matt Angel